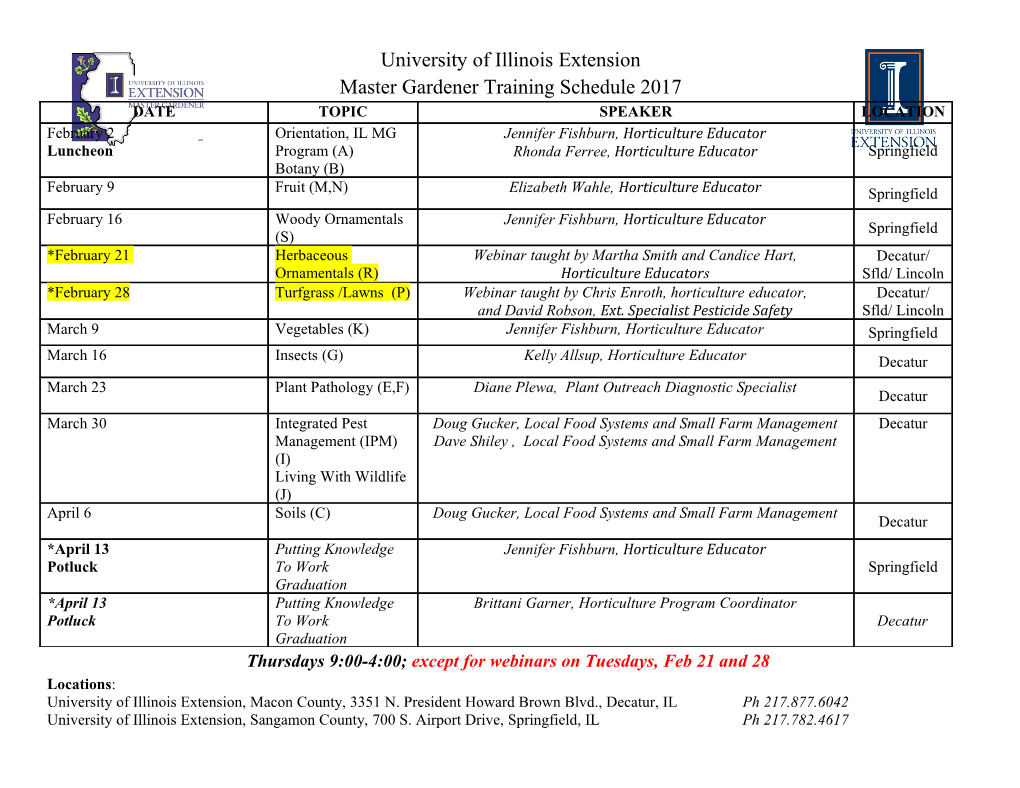
Interpreting past hydroclimate variability from sedimentary records: Challenges & considerations Jessica Tierney The University of Arizona a) Archive types 80oN 60oN borehole 40oN coral 20oN documents glacier ice o 0 hybrid 20oS lake sediment marine sediment o 40 S sclerosponge 60oS speleothem tree 80oS b) Resolution 80oN 50 60oN 20 40oN 10 o 20 N 5 0o 2 20oS 1 Resolution (years) 40oS The farther back in time you go, the 1/2 60oS more important sediments become. 1/6 80oS 1/12 Marine Sediments Lake Sediments Tree Rings Corals c) Temporal Availability First Millennium 600 150 500 100 400 50 0 300 200 # proxies 100 0 200 400 600 800 1000 1200 1400 1600 1800 2000 Year (CE) PAGES2K, 2016, Scientific Data, in prep Figure 1: Spatiotemporal data availability in the PAGES2k database. (a) Geo- graphical distribution, by archive type, coded by color and shape; (b) Temporal resolution in the PAGES2k database, defined here as the median of the spacing between consecutive observation. Shapes as in (a), colors encode the resolution in years (see colorbar); (c) Temporal availability, coded by color as in (a). Some nice features of sediment archives • Long, continuous records • Multiple sensors and proxies can be measured Sedimentary Observations (Proxies) with hydroclimate information • Oxygen isotopes (δ18O) on foraminifera (marine) • Lake levels • Things sensitive to lake levels (Mg/Ca of minerals, δ18O of authigenic carbonate). • δD of leaf waxes • Runoff indicators (Ti concentration, varves) • Microfossil assemblages (pollen, diatoms) Some challenging features of sediment archives • Age uncertainty is usually large • Bioturbation mixes signals • The archive itself adds red noise (e.g. lakes). Interpreting sedimentary archives: an overview 5 200 (a) Climate (b) Smoothed Climate 180 Data on true age model Data on age model #1 160 Data on age model #2 140 0 120 100 80 years before present − 60 −5 Proxy values (offset) True age model Time 40 Age model #1 Age model #2 20 Measured Ages Proxy Observations 0 −10 0 100 200 0 20 40 60 80 100 120 140 160 180 200 Depth Time − Years before present Figure made by Martin Tingley Proper interpretation of hydroclimate data from sediments requires: • Proxy (observation) forward model(s) • An archive model to account for smoothing/reddening • A way to deal with age uncertainty Archive issues: Age Uncertainty The time uncertainty continuum Cross-dated Archives (Tree rings) Layer-counted archives (varves, ice cores, corals) Radiometrically dated - Gaussian (U/Th on speleothems,210Pb in sediments) Radiometric dating - non-Gaussian (14C on sediments) 100 75 50 25 0 Uncertainty in Years R EPORTS Fig. 1. Location and by the planktic foraminifer Globigerina bul- correlation map show- loides reflect the cooler, more nutrient-rich ing the spatial relation waters found along the coast and that season- between variations in al assemblage changes mirror the changes in the abundance of the planktic foraminifer G. upwelling intensity, and thus trade winds, bulloides in the Cariaco over the year (6). Basin (southern Carib- We sampled sediments from box core bean) and SST anoma- PL07-71 BC (395 m water depth), recovered lies in the Atlantic Ocean. from the gentle northern slope of the eastern Mapped correlation co- Cariaco Basin, at continuous 1-mm intervals efficients (r)indicatethe correlationsbetweenbox- (7). The age model for core PL07–71 BC car decadal-averaged G. (Fig. 2) consists of two independently de- bulloides data and histor- rived, mutually supporting age data sets. Age ical SST anomaly data control for the upper 12.6 cm of the core (12)forthetimeperiod (1880 to 1990 A.D.) is based on robust faunal 1856–1990 A.D. Corre- correlations with a nearby box core already lations between G. bul- 210 loides abundance and possessing a well-constrained Pb and varve SST anomaly time series chronology (8). Below a depth of 12.6 cm, are highest (–0.8 Ͻ r Ͻ sediment laminations are less distinct and are Ϫ0.9, significant at the difficult to count reliably. For this reason, 99.9% level) in the North below 12.6 cm (1165 to 1879 A.D.) the age Atlantic region between model is based on a series of accelerator mass 50o and 60oN, indicating that G. bulloides abun- spectrometry (AMS) dates from monospe- dance, and hence trade cific samples of G. bulloides. Radiocarbon- wind–induced upwelling based sedimentation rates for samples imme- on June 1, 2016 over the Cariaco Basin, diately at and below 12.7 cm are the same as are tightly coupled to the 210Pb/varve-based estimates above 12.7 SST anomalies in the cm. The resulting 825-year record has a sam- northern North Atlantic region. ple resolution of about one sample per year near the top of the record, decreasing to one sample per 2.5 years at the base. The recent (1880 to 1990 A.D.) abun- dance record for G. bulloides illustrates sub- Fig. 2. The age model for Cariaco Basin box core PL07-71 BC is based on a 210 14 combination of Pb, varve, and AMS Cchronologies.Fortheupper 12.6 cm http://science.sciencemag.org/ of PL07-71 BC, the age model is based on detailed correlations to PL07-81 BC (A), a nearby box core that is already well dated by 210Pb dating and varve counts (8), through an intermediate box core PL07-64 BC (B); dpm, disintegra- tions per minute. Cores PL07-81 BC and PL07-64 BC lie deeper in the basin and contain identical interbedded turbidites. Varve counts and 210Pb values both indicate that the turbidites are not erosive and that a continuous sediment sequence is present in both cores. Sediments from PL07-64 BC (B) above and between the turbidites were sampled at continuous 1-mm intervals and Downloaded from analyzed for their planktic foraminiferal content. These data were used to develop faunal correlations between PL07-64 BC (B) and PL07-71 BC (C)and to transfer the established chronology from PL07-81 BC (A) to the latter. An example of this type of faunal correlation is shown for the foraminifer Orbulina universa (data shown as a three-point smooth); multiple taxa were used to check and verify the correlations. For sediment in core PL07-71 BC deposited at a depth between 12.6 and 56.4 cm (D), an interval where laminae were too faint to count, the age model is based on a series of 12 AMS 14C Annually resolved ≠ time certain dates measured on monospecific samples of G. bulloides (Table 1). Radiocar- bon dates were converted to the calendar ages shown in (D) using a calibration model for marine samples (35); a local reservoir correction of 420 years, shown to be constant over periods of large climatic change, was used to account for radiocarbon Cariaco Basin Age Model differences between the surface waters and the atmosphere (4). Error bars represent the 1 deviation; the unevenness of the error bars is a function of the marine calibration curve. A third-order regression line passing through both sets of age control points yields an r 2 of 0.98 and was used to assign calendar year ages to all samples in the box core deeper than 12.6 cm. Black et al., 1999, Science 1710 26 NOVEMBER 1999 VOL 286 SCIENCE www.sciencemag.org LAKE CHALLA, KENYA (VARVE-COUNTED) Wolff et al., 2011, Science; Verschuren et al., 2009, Nature, Tierney et al., 2011 QSR 4 published age model 68% uncertainty 3 90% uncertainty ~+/- 1-2 years 2 ~+/- 25-30 years 1 PROXY PC1 0 − 3 −1 −2 −3 1100 1200 1300 1400 1500 1600 1700 1800 1900 YEAR LAKE NAIVASHA, KENYA (14C DATED) Verschuren et al., 2000, Nature 35 dates 68% uncertainty 90% uncertainty 30 published age model 25 20 15 Lake Level (m) 10 5 1200 1400 1600 1800 Year AD OxCal v4.1.7 Bronk Ramsey (2010); r:5 Marine data from Reimer et al (2009); P_Sequence P17815P 1000 Age Modeling Techniques Boundary bottom Simplest approach uses MC R_Date 900.5 OxCal R_Date 840.5 iteration and the constraint of 800 Bronk Ramsey, 1995 R_Date 780.5 Bronk Ramsey,M. Blaauw2008 andsuperposition. J. A. Christen More complex 467 R_Date 700.5 approaches make assumptions R_Date 680.5 R_Date 640.5 about the sedimentation process 600 R_Date 600.5 R_Date 560.5 R_Date 520.5 Position R_Date 460.5 R_Date 420.5 BACON 400 Blaauw & Christen, 2011 R_Date 340.5 R_Date 260.5 R_Date 220.5 cal yr BP 200 R_Date 200.5 R_Date 150.5 R_Date 90.5 R_Date 41.5 0 2000 4000 6000 8000 0 Boundary top 0 50 100 150 200 60000 50000 40000 30000 20000 10000 0 Depth Modelled date (BP) Figure 3: Posterior age–depth model of core RLGH3 (grey), overlaying the calibrated distributions of the individual dates (blue). Grey dots indicate the model’s 95% proba- bility intervals. history, with some fluctuations in accumulation rate at sub-millennial scale (Figure 3). The posteriors for accumulation rate and its variability are comparable to their priors, although the posterior indicates more memory than assumed apriori. As in the previous example of the peat core, the prior used for accumulation rates is su±ciently strong and data cannot provide further information. Little more can be learned from radiocarbon data than what we already know about sediment accumulation in this lake core. What constitutes a reasonable (non-decreasing) age-depth model between 0 and 50 cm, for example? This information is not contained within the radiocarbon data and must be an external input, indeed formally provided by our prior for accumulation rates. There is a clear disagreement between the dates c. 130 to 40 cm depth, which can be attributed to erosion of older organic material from the catchment (Jones et al.
Details
-
File Typepdf
-
Upload Time-
-
Content LanguagesEnglish
-
Upload UserAnonymous/Not logged-in
-
File Pages27 Page
-
File Size-