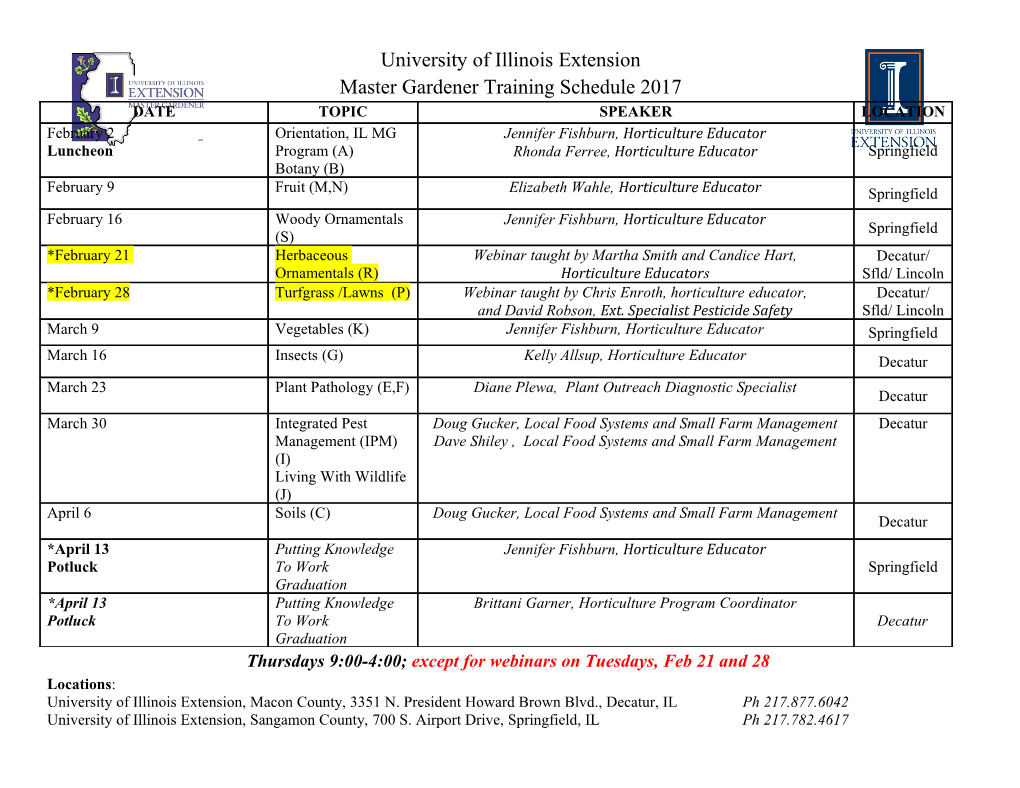
bioRxiv preprint doi: https://doi.org/10.1101/264747; this version posted February 14, 2018. The copyright holder for this preprint (which was not certified by peer review) is the author/funder. All rights reserved. No reuse allowed without permission. 1 Frequency selective encoding of substrate vibrations in the somatosensory cortex 2 3 Mario Prsa and Daniel Huber 4 Department of Basic Neurosciences, University of Geneva, Geneva, Switzerland 5 6 Sensing vibrations that propagate through solid substrates conveys fundamental information about moving objects 7 and other nearby dynamic events. Here we report that neurons responsive to substrate vibrations applied to the 8 mouse forelimb reveal a new way of representing frequency information in the primary somatosensory cortex (S1). In 9 contrast to vibrotactile stimulation of primate glabrous skin, which produces temporally entrained spiking and 10 frequency independent firing rates, we found that mouse S1 neurons rely on a different coding scheme: their spike 11 rates are conspicuously tuned to a preferred frequency of the stimulus. Histology, peripheral nerve block and 12 optogenetic tagging experiments furthermore reveal that these responses are associated with the activation of 13 mechanoreceptors located in deep subdermal tissue of the distal forelimb. We conclude that the encoding of 14 frequency information of substrate‐borne vibrations in the mouse S1 might be analogous to the representation of 15 pitch of airborne sound in auditory cortex. 16 17 Introduction 18 19 Vibrations are ecologically relevant sensory stimuli that can appear in different forms and their spectral content can 20 convey different types of information. For example, the frequency of skin vibrations produced by actively displacing a 21 fingertip across an object provides information about the surface texture. Spikes of S1 neurons responding to skin 22 vibrations of primate fingertips are found to be temporally locked to a preferred phase of the oscillatory cycle, but their 23 rates are reported to be insensitive to stimulus frequency (Harvey et al., 2013; Mountcastle et al., 1969). Frequency 24 dependent spike rate modulations could only be obtained with pulsed vibrations in the flutter range (<40 Hz) (Salinas et 25 al., 2000), in which individual stimulus cycles and not pitch dominate the percept (Saal et al., 2016). The same seems to 26 be true in rodents, where active whisker‐mediated sensation has been advanced as the main modus operandi for 27 collecting somatosensory information from the environment (Diamond et al., 2008); a functional equivalent to active 28 hand palpations in primates. Phase‐locked spiking and frequency independent firing rates of neural responses to passive 29 sinusoidal vibrations of single whiskers have been reported in barrel cortex (Ewert et al., 2008) (but see (Arabzadeh et 30 al., 2004; Arabzadeh et al., 2003)). It therefore seems that in such active sensory systems frequencies are not 31 discriminated based on differences in rates of discharge of cortical neurons. Instead, the proposed neural coding scheme 32 for frequency recognition is based on identifying the dominant phase of the oscillatory stimulus in the cyclically 33 entrained spike trains. 34 35 Different types of vibratory stimuli are generated by external movements and they can be perceived passively. These are 36 transmitted through the solid substrate, and their frequency might indicate the size, speed or distance of, for instance, a 37 nearby conspecific, predator or prey. This passive sensory channel is therefore not primarily adapted to identify object 38 textures or shapes, but rather tuned for gathering essential information about events occurring in the nearby 39 environment. Substrate vibrations propagate away from the surface of contact, typically through the limbs (Hunt, 1961; 40 Hunt and McIntyre, 1960; O’Connell‐Rodwell, 2007) and might therefore activate a broad set of mechanoreceptors 41 distributed along its bones, joints and possibly even in more distant body parts (Bell et al., 1994; Hunt, 1961; Zelena, 42 1994). The involved network of mechanoreceptors might thus be very different from the one probed in experiments 1 bioRxiv preprint doi: https://doi.org/10.1101/264747; this version posted February 14, 2018. The copyright holder for this preprint (which was not certified by peer review) is the author/funder. All rights reserved. No reuse allowed without permission. 1 delivering local skin vibrations at the fingertip, since such vibrations attenuate exponentially with distance and do not 2 seem to propagate beyond the finger (Manfredi et al., 2012). If substrate vibrations indeed rely on a different functional 3 network of mechanoreceptors, might they also have a distinct neural representation of vibration frequency in the 4 cortex? 5 6 To address this question we sought to characterize how neurons in the mouse forelimb representation of the 7 somatosensory cortex (fS1) encode the frequency of substrate vibrations. Using two‐photon calcium imaging and 8 electrophysiology, we found that neurons in the mouse fS1 show spike rates tuned to different stimulus frequencies and 9 lack temporally entrained spiking. They bandpass filter the mechanical stimuli to favor a narrow bandwidth of 10 frequencies where they operate as stimulus feature detectors. We thus propose that the spectral content of structure‐ 11 borne vibrations is extracted at the cortical level using a coding mechanism akin to the one operating in the auditory 12 system for pitch discrimination of airborne sound. This sensory channel therefore appears to be fundamentally different 13 from active skin‐ and whisker‐based somatosensation. 14 15 Results 16 17 Vibration frequency selectivity in somatosensory cortex 18 19 To deliver substrate vibrations to a single forelimb, we trained head‐restrained mice to place their forepaw on a 20 piezoelectric stack actuator and reinforced continuous holding with liquid rewards (Fig. 1A,B, Movie S1, Methods). Pure 21 sinusoidal vibrations (Fig. S1) of different frequencies (100 Hz to 800 Hz) were delivered pseudorandomly to the forepaw 22 at variable inter‐stimulus intervals. To measure the activity of neurons in the contralateral fS1, we expressed the 23 fluorescent Ca2+ indicator GCaMP6f in layer 2/3 neurons using viral vectors and imaged the responses with two‐photon 24 microscopy through a chronic cranial window (Fig. 1C,D). The location of fS1 was identified by intrinsic signal imaging 25 (Fig. 1D). We found that different vibration frequencies reliably evoked transient increases in fluorescence of a subset of 26 imaged neurons (Fig. 1E,F). Since the size of fluorescence transients is positively correlated with the number of spikes in 27 a burst (Chen et al., 2013) we took the fluorescence change relative to baseline (Δf/f0) as a proxy for spike rate. By 28 observing the activity of individual neurons, it became evident that different stimulus frequencies did not evoke equal 29 responses (Fig. 1F), suggesting that fS1 neurons carry information about vibration frequency in the number of spikes 30 they fire. 31 32 To explicitly test the latter hypothesis, we computed the stimulus‐specific information (SSI) as a measure of frequency 33 encoding by the evoked Δf/f0 responses (Butts, 2003; Butts and Goldman, 2006). The majority of responding neurons 34 (>85% of 725 cells, 19 mice, 42 fields of view) were found to be informative about frequency (i.e. had responses with 35 significant SSI, p<0.01, permutation test), which is in stark contrast to the 3% of neurons in the primate S1 responding to 36 fingertip vibrations in the same frequency range (Harvey et al., 2013). To characterize their frequency dependent tuning, 37 mean responses were fit with a descriptive function (Fig. 2A‐H). Strikingly, ≈49% (303 of 621 informative cells) had 38 identifiable tuning curve peaks (Fig. 2A‐D,I) and thus had a preferred frequency of tactile vibration, which is analogous 39 to a fundamental property of auditory cortex neurons: frequency selectivity (Cheung et al., 2001; Issa et al., 2014; 40 Joachimsthaler et al., 2014). The remaining neurons were characterized by frequency dependent response increases‐to‐ 41 plateau or monotonic increases (Fig. 2E‐H,I). 42 2 bioRxiv preprint doi: https://doi.org/10.1101/264747; this version posted February 14, 2018. The copyright holder for this preprint (which was not certified by peer review) is the author/funder. All rights reserved. No reuse allowed without permission. 1 We next assessed the effects of vibration amplitude on frequency tuning. As vibration amplitude was attenuated, a shift 2 of the tuning curves toward higher frequencies was observed (Fig. 2J,K). Also, by reducing the actuator displacement, 3 the range of frequencies that could be generated by the hardware was extended to 1990 Hz. As such, neurons 4 characterized as having increases‐to‐plateau responses at the highest amplitude were found to also be frequency 5 selective when tested over an extended frequency range with smaller displacements (Fig. S2). Based on the descriptive 6 function fits, we identified neuronst tha are tuned to a preferred frequency at any of the three tested displacements. 7 The distributions of their preferred values confirm that fS1 neurons best respond to higher frequencies at smaller 8 stimulus amplitudes (Fig. 2L). The observed amplitude dependent changes in tuning (Fig. 2J,K, Fig. S2) are analogous to 9 how frequency selective responses of auditory cortex neurons change as a function of sound level (Sutter, 2000). 10 11 Frequency selective neurons operate as feature detectors 12 13 What might be the functional relevance of frequency selective tuning of fS1 neurons? Do these neurons signal the 14 presence of a vibratory stimulus at their preferred frequency (i.e. operate as feature detectors) or is their role to 15 differentiate stimuli based on their spectral content (i.e. perform frequency discrimination)? Modeling work has 16 suggested that this question can be answered by analysing what the most informative region of the turning curve is 17 (Butts and Goldman, 2006).
Details
-
File Typepdf
-
Upload Time-
-
Content LanguagesEnglish
-
Upload UserAnonymous/Not logged-in
-
File Pages29 Page
-
File Size-