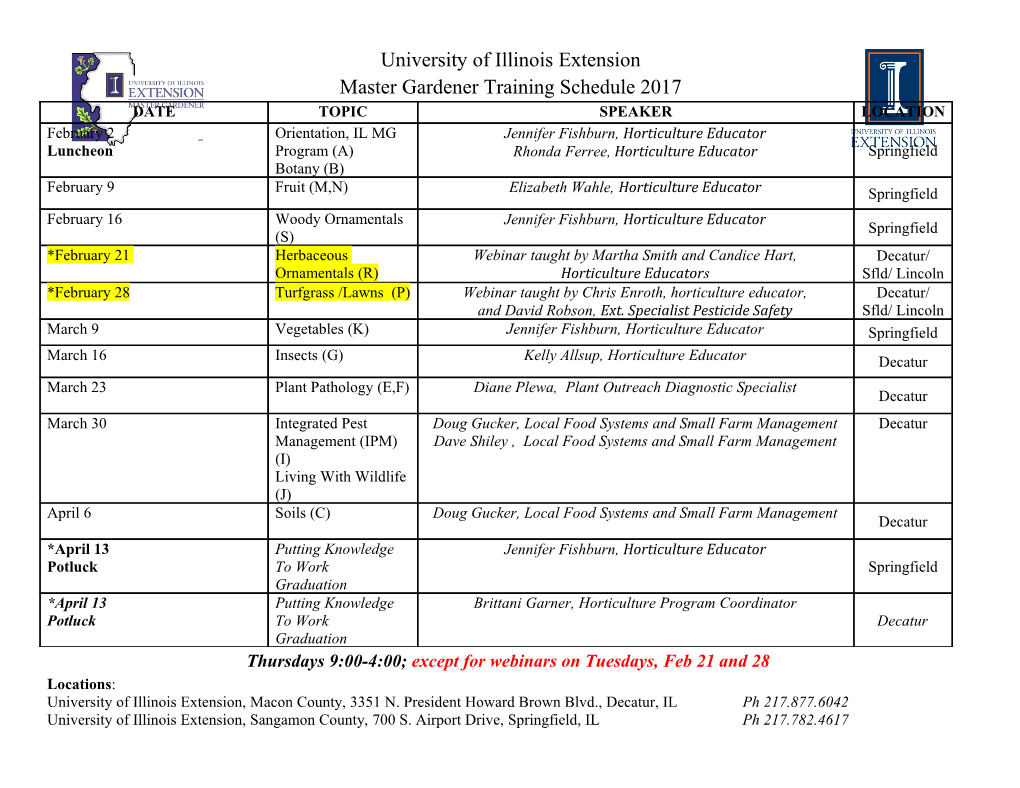
Amplification of RNA by an RNA polymerase ribozyme David P. Horninga,b and Gerald F. Joycea,b,1 aDepartment of Chemistry, The Scripps Research Institute, La Jolla, CA 92037; and bSkaggs Institute for Chemical Biology, The Scripps Research Institute, La Jolla, CA 92037 Contributed by Gerald F. Joyce, June 23, 2016 (sent for review May 17, 2016; reviewed by Ronald R. Breaker and Peter J. Unrau) In all extant life, genetic information is stored in nucleic acids that polymerization, this approach does not select directly for chemical are replicated by polymerase proteins. In the hypothesized RNA bond formation, but rather for the efficient and accurate transfer world, before the evolution of genetically encoded proteins, ances- of functional information from template to cRNA. A polymerase tral organisms contained RNA genes that were replicated by an RNA variant isolated after 24 rounds of evolution exhibited dramatically polymerase ribozyme. In an effort toward reconstructing RNA-based improved activity and template generality, enabling both the syn- life in the laboratory, in vitro evolution was used to improve thesis of structurally complex RNAs and the replication of short dramatically the activity and generality of an RNA polymerase RNA sequences in a protein-free form of the PCR. This work ribozyme by selecting variants that can synthesize functional RNA demonstrates that the replication and expression of genetic mate- molecules from an RNA template. The improved polymerase ribozyme rial can be accomplished with RNA alone. The prospect for further is able to synthesize a variety of complex structured RNAs, including improvement of these activities suggests a practical route toward aptamers, ribozymes, and, in low yield, even tRNA. Furthermore, the the development of RNA-based life in the laboratory. polymerase can replicate nucleic acids, amplifying short RNA templates by more than 10,000-fold in an RNA-catalyzed form of the PCR. Thus, Results the two prerequisites of Darwinian life—the replication of genetic in- Evolution Based on Aptamer Completion. An engineered form of the formation and its conversion into functional molecules—can now be class I polymerase ribozyme [wild type (WT); Fig. S1] was constructed accomplished with RNA in the complete absence of proteins. by combining known advantageous features, including a large de- letion within the 3′-terminal domain; a 5′-terminal sequence tag that self-replication | ribozyme | RNA enzyme | PCR | in vitro evolution binds to a complementary region of the template; and a set of activity-enhancing mutations (14, 16). Random mutations then BIOCHEMISTRY he core informational processes of Darwinian evolution are were introduced throughout the molecule at a frequency of 10% Tthe replication of genes and their expression as functional per nucleotide position to generate a population of 1014 distinct molecules, which in modern biology require the action of protein variants to initiate the in vitro evolution process. During each enzymes. Features common to all extant life suggest the exis- round of evolution (Fig. 1A), an RNA primer was covalently tence of an RNA world where the replication and expression of attached to the 5′ end of the polymerase and nucleotide exten- genetic information depended on RNA enzymes rather than sion reactions were performed using a separate RNA template genetically encoded proteins (1, 2). Accordingly, substantial ef- and the four nucleoside triphosphates (NTPs). forts have been directed toward reconstructing RNA life via the Two forms of selection pressure were applied to the population protein-free replication of RNA (3, 4). RNA-joining ribozymes to obtain more active polymerases. First, the polymerase was have been modified to assemble new copies of themselves from challenged to extend the attached primer to complete a 3′-trun- smaller RNA substrates (5), in one case achieving self-replica- catedRNAaptamer,enablingselectionbasedonbindingofthe tion with exponential growth (6). However, these self-replicating completed aptamer to its cognate ligand. Both the cyanocobalamin ribozymes require complex oligonucleotide substrates, which (17) and GTP (18) aptamers were used to provide varied sequence B limits their ability to synthesize functional RNA molecules other contexts that are largely intolerant of mutation (Fig. 1 ), thus im- than additional copies of themselves, which in turn limits their posing selection pressure for both sequence generality and accuracy. ability to express and evolve functions beyond self-replication. A more general solution is offered by the template-directed Significance polymerization of RNA monomers, the mechanism used in modern biology for the synthesis of DNA and RNA. RNA- Darwinian life requires the ability to replicate genotypes and templated RNA polymerization has been demonstrated both express phenotypes. Although all extant life relies on protein nonenzymatically (7, 8) and with a variety of natural and syn- enzymes to accomplish these tasks, life in the ancestral RNA thetic ribozymes (9–11). Although none of these systems have world would have used only RNA enzymes. Here, we report been able to replicate RNA exponentially, extensive copying of the in vitro evolution of an improved RNA polymerase ribo- RNA templates has been achieved with evolved variants of the zyme that is able to synthesize structured functional RNAs, class I RNA polymerase ribozyme. This ribozyme can synthesize including aptamers and ribozymes, and replicate short RNA tandem RNA repeats over 100 nt long and even complete a sequences in a protein-free form of the PCR. Thus, the repli- substantial portion of a small endonuclease ribozyme (12–15). cation of RNA and the expression of functional RNA can be However, the polymerase strongly prefers cytidine-rich templates accomplished with RNA alone. Combining and improving these that lack any secondary structure and has much more limited activities may enable the self-sustained evolution of RNA and activity in other contexts (15). These limitations preclude the offers a potential route to a synthetic form of RNA life. synthesis of most functional RNAs, which often are highly Author contributions: D.P.H. and G.F.J. designed research; D.P.H. performed research; structured, and the replication of RNA, which requires the re- D.P.H. and G.F.J. analyzed data; and D.P.H. and G.F.J. wrote the paper. ciprocal synthesis of both an RNA template and its complement. Reviewers: R.R.B., Yale University; and P.J.U., Simon Fraser University. Template restrictions might be eased by using in vitro evolu- Conflict of interest statement: R.R.B. and G.F.J. appeared as coauthors of a paper published as tion to select directly for the synthesis of functional RNAs from Breaker RR, Joyce GF (2014) The expanding view of RNA and DNA function. Chem Biol 21(9):1059– “difficult” templates. In the present study, an engineered form of 1065. This paper was a Perspective commemorating the 20th anniversary of the journal. the class I polymerase was selected for its ability to synthesize 1To whom correspondence should be addressed. Email: [email protected]. functional RNA aptamers from complementary templates. In This article contains supporting information online at www.pnas.org/lookup/suppl/doi:10. contrast to previous in vitro evolution efforts directed at RNA 1073/pnas.1610103113/-/DCSupplemental. www.pnas.org/cgi/doi/10.1073/pnas.1610103113 PNAS Early Edition | 1of6 Downloaded by guest on September 24, 2021 A 1 2 7 3 6 5 4 ACAAG - 3´ B G G U CGA U U G G A G U U G C G U 5´- GGG AAC G G C G C U C 5´- GGA ACGC G U GGC CA 3´- CCC UG CA A G U G C G G U AU AG A C C A CC A AC U C AA CGA A A A C G C A C A C C C A C A A GG C U A U A G C G C C CG A U A U C U A CAA G U C A A C U A U C A A GC G C G G A U GUU C A G U U C G A A G C A GC C A A 3´- C A G U AAC GC U CG G 5´- N N 5´- A G UCA UUG C G C A C U C G UA A C 3´ GC CAAG – AGACAAAU UA UG A A A C A U G U G CCAC C GGAG GGGA C G G C A C A A G GUGGC U UCC CCU GC C G U Fig. 1. In vitro evolution of RNA polymerase ribozymes. (A) Selective amplification of ribozymes that extend a tethered RNA primer (magenta) on a separate RNA template (brown) to complete a 3′-truncated aptamer. (1) Attachment of the primer to the ribozyme via a photocleavable linker; (2) hybridization of the primer to the template; (3) extension of the primer by polymerization of NTPs (cyan); (4) capture of full-length materials by binding the aptamer portion to its immobilized ligand (green); (5) photocleavage to release the ribozyme portion; (6) reverse transcription and PCR amplification of the released ribozyme; and (7) transcription to generate progeny ribozymes. (B) Sequence and secondary structure of RNA aptamers that bind either cyanocobalamin (Left) or GTP (Right). (C) Sequence and secondary structure of the 24-3 polymerase. Red circles indicate mutations relative to WT, with color intensity indicating mutations that were universal (dark), common (medium), or sporadic (light) among the clones that were sequenced. Second, a gel-shift selection procedure was used to ensure the Properties of the 24-3 Polymerase. The rate of polymerase-catalyzed polymerase extension products had reached full length, as reflected nucleotide addition to a template-bound primer was measured by their corresponding mobility in a denaturing polyacrylamide gel. using a cytidine-rich template of 11 nt, which has been used ex- Twenty-four rounds of in vitro evolution were carried out tensively to demonstrate the prowess of the class I polymerase (12– (Table S1), progressively increasing the selection stringency by 15).
Details
-
File Typepdf
-
Upload Time-
-
Content LanguagesEnglish
-
Upload UserAnonymous/Not logged-in
-
File Pages6 Page
-
File Size-