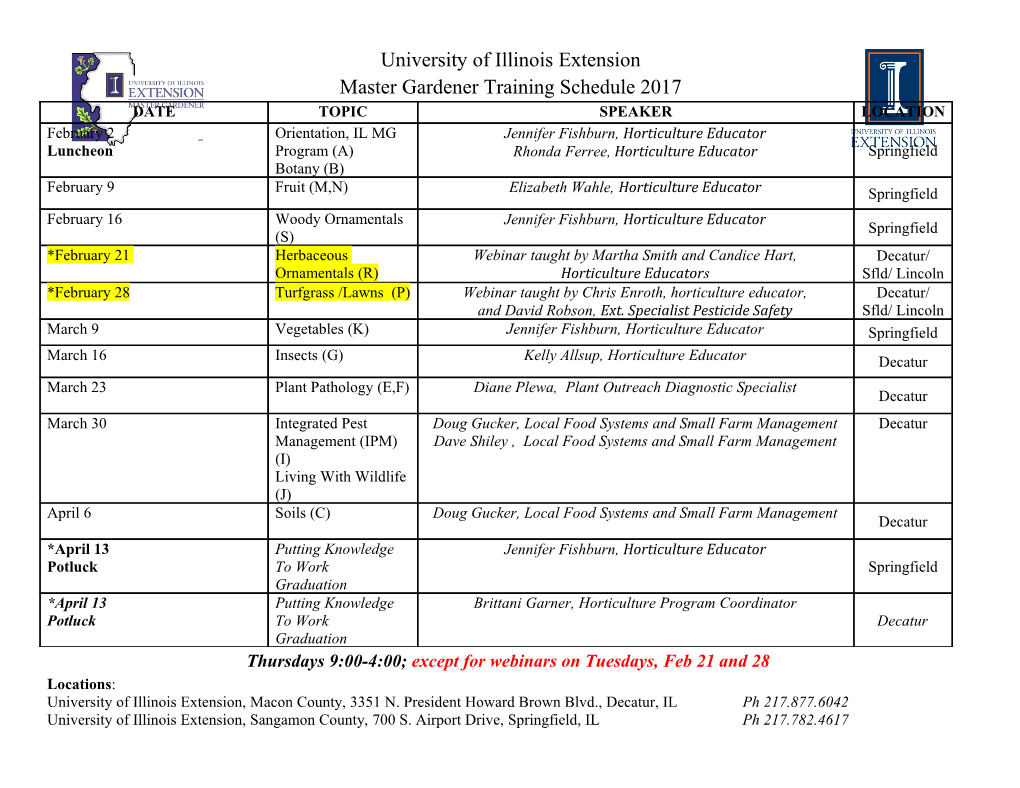
LOCALIZATION AND STANDARD MODULES FOR REAL SEMISIMPLE LIE GROUPS II: IRREDUCIBILITY, VANISHING THEOREMS AND CLASSIFICATION HENRYK HECHT, DRAGAN MILICIˇ C,´ WILFRIED SCHMID, AND JOSEPH A. WOLF Contents 1. Introduction 1 2. Generalities on intertwining functors 1 3. Supports and n-homology 13 4. Calculations for sl(2; C) 20 5. Some results on root systems with involution 32 6. K-orbits in the flag variety 38 7. Intertwining functors and standard Harish-Chandra sheaves 48 8. Irreducibility of standard Harish-Chandra sheaves 57 9. Geometric classification of irreducible Harish-Chandra modules 65 10. Decomposition of global sections of standard Harish-Chandra sheaves 71 11. n-homology of Harish-Chandra modules 75 12. Tempered Harish-Chandra modules 79 13. Quasispherical Harish-Chandra modules 81 References 84 1. Introduction 2. Generalities on intertwining functors ∗ Let θ be a Weyl group orbit in h . We consider the category M(Uθ) of Uθ- modules. For each λ 2 θ we also consider the category Mqc(Dλ) of (quasicoherent) Dλ-modules. Assigning to a Dλ-module V its global sections Γ(X; V) defines a functor Γ : Mqc(Dλ) −! M(Uθ). Its left adjoint is the localization functor ∆λ : M(Uθ) −! Mqc(Dλ) given by ∆λ(V ) = Dλ ⊗Uθ V . Let Σλ be the set of roots integral with respect to λ, i.e., Σλ = fα 2 Σ j αˇ(λ) 2 Zg: Then the subgroup Wλ of the Weyl group W generated by the reflections with respect to the roots from Σλ is equal to Wλ = fw 2 W j wλ − λ 2 Q(Σ)g; ∗ where Q(Σ) is the root lattice of Σ in h ([7], Ch. VI, §2, Ex. 2). Let Πλ be the set of simple roots in the root system Σλ attached to the set of positive roots + + Σλ = Σλ \ Σ . Denote by `λ : Wλ −! Z+ the corresponding length function. 2020 Mathematics Subject Classification. Primary 22E46. 1 2 H. HECHT, D. MILICIˇ C,´ W. SCHMID, AND J. A. WOLF We say that λ is antidominant if αˇ(λ) is not a strictly positive integer for any α 2 Σ+. For arbitrary λ we define n(λ) = minf`λ(w) j wλ is antidominant, w 2 Wλg: The following result was established in [4] and [11]. Theorem 2.1. Let λ 2 h∗ and θ = W · λ. Then (i) The right cohomological dimension of Γ: Mqc(Dλ) −! M(Uθ) is ≤ n(λ). (ii) The left cohomological dimension of ∆λ : M(Uθ) −! Mqc(Dλ) is finite if and only if λ is regular. (iii) If λ is regular, the left cohomological dimension of ∆λ is ≤ n(λ). Consider the derived category D(Uθ) of complexes of Uθ-modules and the de- rived category D(Dλ) of complexes of Dλ-modules. By (i), there exists the de- rived functor RΓ: D(Dλ) −! D(Uθ). This functor also induces functors be- tween the corresponding full subcategories of bounded complexes. On the other hand, for arbitrary λ, there exists also the derived functor of localization functor − − L∆λ : D (Uθ) −! D (Dλ) between derived categories of complexes bounded from above. If λ is regular, the left cohomological dimension of ∆λ is finite by (ii), and L∆λ extends to the derived functor between D(Dλ) and D(Uθ). Moreover, it maps bounded complexes into bounded complexes. We have the following result [4]. ∗ b b Theorem 2.2. Let λ 2 h be regular and θ = W ·λ. Then RΓ: D (Dλ) −! D (Uθ) b b and L∆λ : D (Uθ) −! D (Dλ) are mutually quasiinverse equivalences of categories. b This implies, in particular, that for any two λ, µ 2 θ, the categories D (Dλ) and b D (Dµ) are equivalent. This equivalence is given by the functor L∆µ ◦ RΓ from b b D (Dλ) into D (Dµ). In this section we describe a functor, defined in geometric terms, which is (under certain conditions) isomorphic to this functor. This is the intertwining functor of Beilinson and Bernstein [4]. Most of the following results on the intertwining functors are due to Beilinson and Bernstein and were announced in [4], [2]. Complete details can be found in [14]. We start with some geometric remarks. Define the action of G = Int(g) on X ×X by g · (x; x0) = (g · x; g · x0) for g 2 G and (x; x0) 2 X × X. The G-orbits in X × X can be parametrized in the following way. First we introduce a relation between Borel subalgebras in g. Let b and b0 be two Borel subalgebras in g, n and n0 their nilpotent radicals and N and N 0 the corresponding subgroups of G. Let c be a Cartan subalgebra of g contained in b \ b0. Denote by R the root system of (g; c) in c∗ and by R+ the set of positive roots determined by b. This determines a specialization of the Cartan triple (h∗; Σ; Σ+) into (c∗; R; R+) [12]. On the other hand, b0 determines another set of positive roots in R, which corresponds via this specialization to w(Σ+) for some uniquely determined w 2 W . The element w 2 W does not depend on the choice of c, and we say that b0 is in relative position w with respect to b. Let 0 Zw = f(x; x ) 2 X × X j bx0 is in the relative position w with respect to bxg IRREDUCIBILITY, VANISHING THEOREMS AND CLASSIFICATION 3 for w 2 W . Then the map w −! Zw is a bijection of W onto the set of G-orbits in X × X, hence the sets Zw, w 2 W , are smooth subvarieties of X × X. Denote by p1 and p2 the projections of Zw onto the first and second factor in the product X × X, respectively. The fibrations pi : Zw −! X, i = 1; 2, are locally trivial with fibres isomorphic to `(w)-dimensional affine spaces. Hence, they are are affine morphisms. Let ΩZwjX be the invertible OZw -module of top degree relative differential forms for the projection p1 : Zw −! X. Let Tw be its inverse. Since the tangent space at 0 (x; x ) 2 Zw to the fibre of p1 can be identified with nx=(nx \ nx0 ), and ρ − wρ is the sum of roots in Σ+ \ (−w(Σ+)), we see that ∗ Tw = p1(O(ρ − wρ)): It is easy to check that p1 p2 Tw (Dwλ) = (Dλ ) ([12], Appendix A). Since the morphism p2 : Zw −! X is a surjective submersion, + p2 p2 is an exact functor from Mqc(Dλ) into Mqc((Dλ) ). Twisting by Tw defines an exact functor V −! T ⊗ p+(V) from M(D ) into M ((D )p1 ) ([12], A.3.3.1). w OZw 2 λ qc wλ Therefore, we have a functor V· −! T ⊗ p+(V·) from Db(D ) into Db((D )p1 ). w OZw 2 λ wλ b p1 b Composing it with the direct image functor Rp1+ : D ((Dwλ) ) −! D (Dwλ), we b b get the functor Jw : D (Dλ) −! D (Dwλ) by the formula J (V·) = Rp (T ⊗ p+(V·)) w 1+ w OZw 2 · b for any V 2 D (Dλ). Let V 2 Mqc(Dλ). Since p1 is an affine morphism with i `(w)-dimensional fibres, it follows that H (Jw(D(V))) vanishes for i < −`(w) and i > 0. Moreover, the functor I (V) = R0p (T ⊗ p+(V)) w 1+ w OZw 2 from Mqc(Dλ) into Mqc(Dwλ) is right exact. This is the intertwining functor (attached to w 2 W ) between Mqc(Dλ) and Mqc(Dwλ). One knows that Jw is actually the left derived functor LIw of Iw ([4], [14]); moreover, ∗ b b Proposition 2.3. Let w 2 W and λ 2 h . Then LIw = Jw : D (Dλ) −! D (Dwλ) is an equivalence of categories. For a weight ν 2 P (Σ) we denote by O(ν) the corresponding homogeneous in- vertible OX -module. From the construction of the intertwining functors one can easily check that they behave nicely with respect to twists by homogeneous invert- ible OX -modules: Lemma 2.4. Let w 2 W , λ 2 h∗ and ν 2 P (Σ). Then · · LIw(V (ν)) = LIw(V )(wν) · b for any V 2 D (Dλ). Intertwining functors satisfy a natural \product formula". To formulate it we need some additional geometric information on G-orbits in X × X. Let w; w0 2 0 0 W . Denote by p1 and p2 the projections of Zw into X, and by p1 and p2 the corresponding projections of Zw0 into X. Let Zw0 ×X Zw be the fibre product of Zw0 0 0 and Zw with respect to the morphisms p2 and p1. Denote by q : Zw0 ×X Zw −! Zw0 and q : Zw0 ×X Zw −! Zw the corresponding projections to the first, resp. second 0 0 factor. Finally, the morphisms p1 ◦q : Zw0 ×X Zw −! X and p2 ◦q : Zw0 ×X Zw −! 4 H. HECHT, D. MILICIˇ C,´ W. SCHMID, AND J. A. WOLF X determine a morphism r : Zw0 ×X Zw −! X×X. Therefore, we have the following commutative diagram. X × X O r Zw0 ×X Zw q0 q { # Zw0 Zw 0 p 1 p2 0 p1 p 2 ~ XXX$ { All morphisms in the diagram are G-equivariant. From the construction it follows that the image of r is contained in Zw0w, and by the G-equivariance of r it is a 0 surjection of Zw0 ×X Zw onto Zw0w. Assume in addition that w; w 2 W are such 0 0 that `(w w) = `(w ) + `(w). Then r : Zw0 ×X Zw −! Zw0w is an isomorphism.
Details
-
File Typepdf
-
Upload Time-
-
Content LanguagesEnglish
-
Upload UserAnonymous/Not logged-in
-
File Pages85 Page
-
File Size-