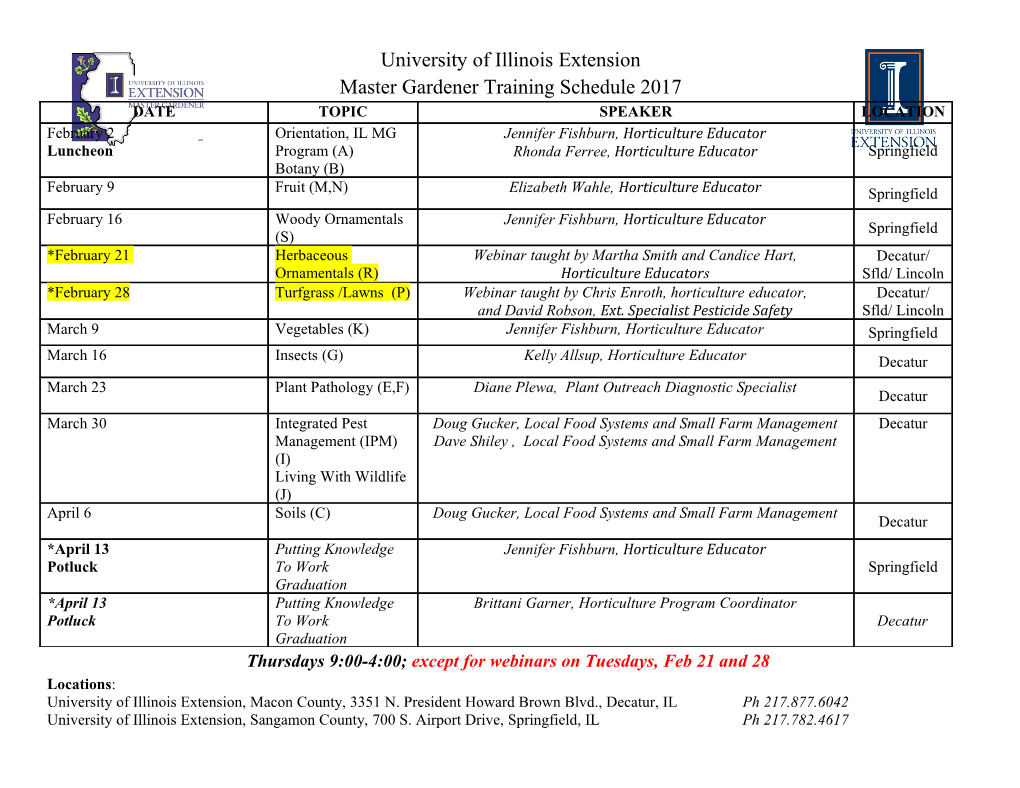
Living Rev. Solar Phys., 12, (2015), 2 ,)6).' 2%6)%73 DOI 10.1007/lrsp-2015-2 INSOLARPHYSICS The FIP and Inverse FIP Effects in Solar and Stellar Coronae J. Martin Laming Code 7684 Naval Research Laboratory Washington DC 20375, USA email: [email protected] Accepted: 22 July 2015 Published: 9 September 2015 Abstract We review our state of knowledge of coronal element abundance anomalies in the Sun and stars. We concentrate on the first ionization potential (FIP) effect observed in the solar corona and slow-speed wind, and in the coronae of solar-like dwarf stars, and the \inverse FIP" effect seen in the corona of stars of later spectral type; specifically M dwarfs.These effects relate to the enhancement or depletion, respectively, in coronal abundance with respect to photospheric values of elements with FIP below about 10 eV. They are interpreted in terms of the ponderomotive force due to the propagation and/or reflection of magnetohydrodynamic waves in the chromosphere. This acts on chromospheric ions, but not neutrals, and so can lead to ion-neutral fractionation. A detailed description of the model applied to closed magnetic loops, and to open field regions is given, accounting for the observed difference in solar FIP fractionation between the slow and fast wind. It is shown that such a model can also account for the observed depletion of helium in the solar wind. The helium depletion is sensitive to the chromospheric altitude where ion-neutral separation occurs, and the behavior of the helium abundance in the closed magnetic loop strongly suggests that the waves have a coronal origin. This, and other similar inferences may be expected to have a strong bearing on theories of solar coronal heating. Chromospheric waves originating from below as acoustic waves mode convert, mainly to fast-mode waves, can also give rise to ion-neutral separation. Depending on the geometry of the magnetic field, this can result in FIP or Inverse FIP effects. We argue that such configurations are more likely to occur in later-type stars (known to have stronger field in any case), and that this explains the occurrence of the Inverse FIP effect in M dwarfs. We conclude witha discussion of possible directions for future work. Keywords: Stars: coronae, Sun: abundances, Sun: chromosphere, Sun: corona, Turbulence, Waves © The Author(s). This article is distributed under a Creative Commons Attribution 4.0 International License. http://creativecommons.org/licenses/by/4.0/ Imprint / Terms of Use Living Reviews in Solar Physics is a peer-reviewed open access journal published by the Springer International Publishing AG, Gewerbestrasse 11, 6330 Cham, Switzerland. ISSN 1614-4961. This article is distributed under the terms of the Creative Commons Attribution 4.0 International License (http://creativecommons.org/licenses/by/4.0/), which permits unrestricted use, dis- tribution, and reproduction in any medium, provided you give appropriate credit to the original author(s) and the source, provide a link to the Creative Commons license, and indicate if changes were made. Figures that have been previously published elsewhere may not be reproduced without consent of the original copyright holders. J. Martin Laming, \The FIP and Inverse FIP Effects in Solar and Stellar Coronae", Living Rev. Solar Phys., 12, (2015), 2. DOI 10.1007/lrsp-2015-2. Article Revisions Living Reviews supports two ways of keeping its articles up-to-date: Fast-track revision. A fast-track revision provides the author with the opportunity to add short notices of current research results, trends and developments, or important publications to the article. A fast-track revision is refereed by the responsible subject editor. If an article has undergone a fast-track revision, a summary of changes will be listed here. Major update. A major update will include substantial changes and additions and is subject to full external refereeing. It is published with a new publication number. For detailed documentation of an article's evolution, please refer to the history document of the article's online version at http://dx.doi.org/10.1007/lrsp-2015-2. Contents 1 Introduction 5 2 Solar Photospheric Composition 6 2.1 Review ........................................... 6 2.2 Helioseismology ...................................... 6 3 The Solar FIP Effect: Overview 9 4 Stellar FIP and Inverse FIP Effects 14 5 Early Theoretical Models: Overview 19 5.1 Diffusion models and variations ............................. 19 5.2 Thermoelectric driving .................................. 20 5.3 Chromospheric reconnection ............................... 21 5.4 Ion cyclotron wave heating ............................... 21 5.5 Summary ......................................... 22 6 The Ponderomotive Force Model 23 6.1 Overview ......................................... 23 6.2 The ponderomotive force ................................. 25 6.3 Chromospheric model .................................. 26 6.4 The Alfv´en wave transport equations .......................... 28 6.5 Fractionation ....................................... 30 6.6 Compressional chromospheric waves .......................... 32 6.6.1 Introduction ................................... 32 6.6.2 Parametric generation by Alfv´enwaves .................... 32 6.6.3 Fast-mode waves from above .......................... 33 6.6.4 P-modes from below ............................... 33 7 Results and Interpretation 35 7.1 Closed loop ........................................ 35 7.2 Open field ......................................... 39 7.3 The helium abundance .................................. 43 7.4 Significance of coronal Alfv´enwaves .......................... 44 7.5 Modeling the inverse FIP effect ............................. 47 7.6 Saturation ......................................... 53 8 Conclusions & Future Work 54 References 58 List of Tables 1 Recommended Solar Photospheric Abundances of Common Elements . 7 2 List of FIP Bias Assessments for Main-Sequence Stars . 15 3 FIP Fractionations in Closed Magnetic Field ..................... 38 4 FIP Fractionations in Open Magnetic Field ...................... 42 5 FIP and Inverse FIP Effects ............................... 52 The FIP and Inverse FIP Effects in Solar and Stellar Coronae 5 1 Introduction Working during the early years of solar UV and X-ray spectroscopy, Pottasch (1963) found evi- dence for significantly higher abundances of Mg, Si, and Fe in the low solar corona thaninthe photosphere, and concluded, somewhat reluctantly that \the chemical composition in the solar atmosphere differs from the photosphere to the corona". These elements, Mg, Si, and Fe,areall elements with first ionization potential (FIP) less than 10 eV, now known to be routinely enhanced in abundance in the corona with respect to photospheric values, a phenomenon that has become known as the \FIP effect”. High FIP elements such as O, Ne, and He, have much smaller abundance enhancements, or even abundance depletions in the corona. Although the possibility of elemental fractionation between the solar photosphere and corona only really began to be taken seriously in the mid 1980s, with the publication of influential reviews by Meyer (1985a,b), recognizing the work of Pottasch (1963) almost fifty years ago makes the problem of understanding the FIP effect nearly as old as that of coronal heating. In fact, modern models of the effect to be discussed in detail below, in which the fractionation is driven by the ponderomotive force of Alfv´enwaves, make an intimate connection between the abundance anomaly and coronal heating mechanisms, such that the FIP effect may yield several important insights into the nature of the latter. This isatheme we will develop throughout this review. The solar FIP effect manifests itself in several modes of observation. Spectroscopy, as pioneered by Pottasch (1963) and extensively reviewed at intervals over the last 20 years (e.g., Feldman, 1992; Feldman and Laming, 2000; Feldman and Widing, 2003; Saba, 1995), reveals the composition primarily of the \closed loop" corona. Meyer (1985a,b) also considered elemental abundances measured in situ in the solar wind, and in solar energetic particle events, which flow out along open magnetic field lines. Observations with Ulysses, the first mission to fly over the solarpolar regions (Wenzel et al., 1992) revealed FIP fractionation varying with wind speed (Zurbuchen et al., 1999; von Steiger et al., 2000). Slow speed solar wind had abundances resembling those in the closed loop solar corona, whereas high speed wind from polar coronal holes had a much lower level of FIP fractionation. With the advent of the Solar and Heliospheric Observatory (SOHO), a more coherent observational picture began to emerge. Coronal holes, the source of the relatively unfractionated fast solar wind, were themselves shown to have similar abundances to the wind emanating from them. The lower latitude closed field corona was also shown to have FIP fractionated plasma, similar to the slow speed solar wind. Schmelz et al. (2012) give a modern view of coronal element abundances derived from these various solar physics sources, coronal spectroscopy, solar wind and solar energetic particles. Astrophysical EUV and X-ray spectroscopy, made possible by the 1990's launches of the Extreme Ultraviolet Explorer (EUVE), the Advanced Satellite for Cosmology and Astrophysics (ASCA), Chandra and XMM-Newton, allowed coronal abundances in stars to be measured for the first time. Again, nearly two decades after the first
Details
-
File Typepdf
-
Upload Time-
-
Content LanguagesEnglish
-
Upload UserAnonymous/Not logged-in
-
File Pages76 Page
-
File Size-