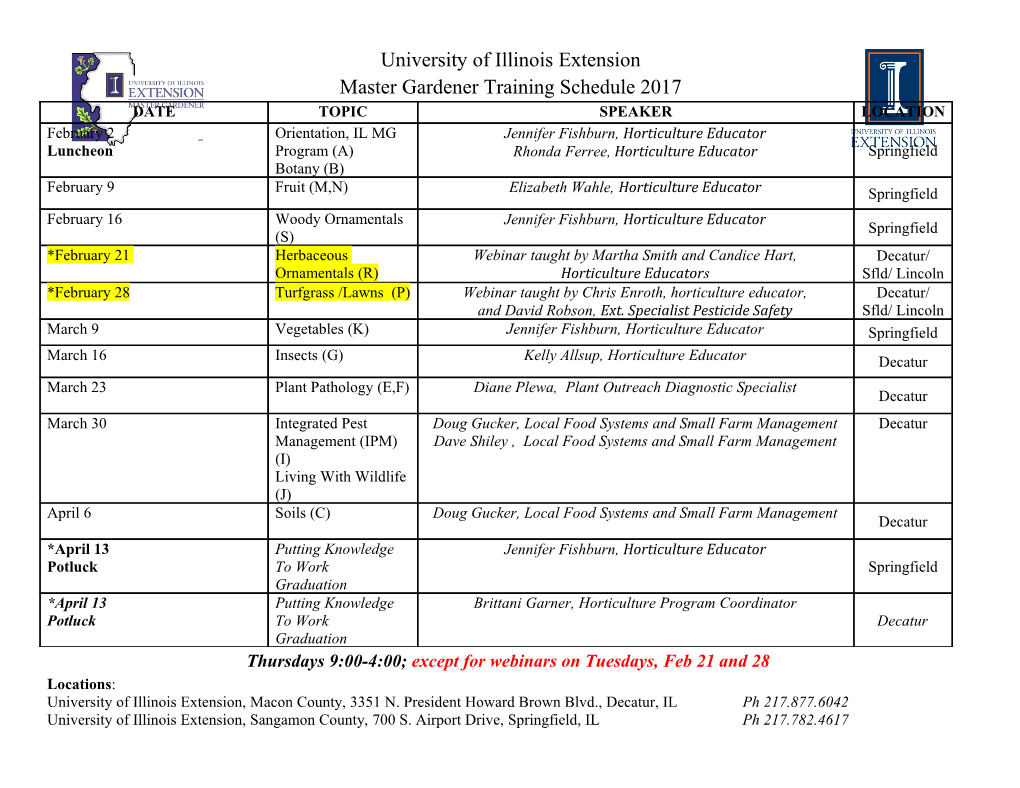
Physical Processes in the Interstellar Medium Ralf S. Klessen and Simon C. O. Glover Abstract Interstellar space is filled with a dilute mixture of charged particles, atoms, molecules and dust grains, called the interstellar medium (ISM). Understand- ing its physical properties and dynamical behavior is of pivotal importance to many areas of astronomy and astrophysics. Galaxy formation and evolu- tion, the formation of stars, cosmic nucleosynthesis, the origin of large com- plex, prebiotic molecules and the abundance, structure and growth of dust grains which constitute the fundamental building blocks of planets, all these processes are intimately coupled to the physics of the interstellar medium. However, despite its importance, its structure and evolution is still not fully understood. Observations reveal that the interstellar medium is highly tur- bulent, consists of different chemical phases, and is characterized by complex structure on all resolvable spatial and temporal scales. Our current numerical and theoretical models describe it as a strongly coupled system that is far from equilibrium and where the different components are intricately linked to- gether by complex feedback loops. Describing the interstellar medium is truly a multi-scale and multi-physics problem. In these lecture notes we introduce the microphysics necessary to better understand the interstellar medium. We review the relations between large-scale and small-scale dynamics, we con- sider turbulence as one of the key drivers of galactic evolution, and we review the physical processes that lead to the formation of dense molecular clouds and that govern stellar birth in their interior. Universität Heidelberg, Zentrum für Astronomie, Institut für Theoretische Astrophysik, Albert-Ueberle-Straße 2, 69120 Heidelberg, Germany e-mail: [email protected],[email protected] 1 Contents Physical Processes in the Interstellar Medium ................ 1 Ralf S. Klessen and Simon C. O. Glover 1 Introduction..........................................4 2 Composition of the ISM................................8 2.1 Gas..........................................8 2.2 Dust......................................... 11 2.3 Interstellar radiation field....................... 13 2.4 Cosmic rays................................... 18 3 Heating and cooling of interstellar gas................... 21 3.1 Optically-thin two-level atom.................... 21 3.2 Effects of line opacity........................... 26 3.3 Multi-level systems............................. 29 3.4 Atomic and molecular coolants in the ISM........ 31 3.5 Gas-grain energy transfer....................... 41 3.6 Computing the dust temperature................ 45 3.7 Photoelectric heating........................... 47 3.8 Other processes responsible for heating........... 51 4 ISM Turbulence....................................... 56 4.1 Observations.................................. 56 4.2 Simple theoretical considerations................. 67 4.3 Scales of ISM Turbulence....................... 73 4.4 Decay of ISM turbulence........................ 77 4.5 Sources of ISM turbulence: gravity and rotation.... 78 4.6 Sources of ISM turbulence: stellar feedback........ 84 5 Formation of molecular clouds.......................... 91 5.1 Transition from atomic to molecular gas.......... 91 5.2 Importance of dust shielding.................... 102 5.3 Molecular cloud formation in a galactic context.... 105 6 Star formation........................................ 111 6.1 Molecular cloud cores as sites of star formation.... 111 6.2 Statistical properties of stars and star clusters..... 117 3 4 Introduction 6.3 Gravoturbulent star formation................... 121 6.4 Theoretical models for the origin of the IMF...... 124 6.5 Massive star formation......................... 138 6.6 Final stages of star and planet formation.......... 140 7 Summary............................................ 142 References................................................. 147 1 Introduction Understanding the physical processes that govern the dynamical behavior of the interstellar medium (ISM) is central to much of modern astronomy and astrophysics. The ISM is the primary galactic repository out of which stars are born and into which they deposit energy, momentum and enriched material as they die. It constitutes the anchor point of the galactic matter cycle, and as such is the key to a consistent picture of galaxy formation and evolution. The dynamics of the ISM determines where and when stars form. Similarly, the properties of the planets and planetary systems around these stars are intimately connected to the properties of their host stars and the details of their formation process. When we look at the sky on a clear night, we can notice dark patches of obscuration along the band of the Milky Way. These are clouds of dust and gas that block the light from distant stars. With the current set of telescopes and satellites we can observe dark clouds at essentially all frequencies possi- ble, ranging from low-energy radio waves all the way up to highly energetic γ-rays. We have learned that all star formation occurring in the Milky Way and other galaxies is associated with these dark clouds that mostly consist of cold molecular hydrogen and dust. In general, these dense clouds are embed- ded in and dynamically connected to the larger-scale and less dense atomic component. Once stellar birth sets in, feedback becomes important. Massive stars emit copious amounts of ionizing photons and create bubbles of hot ionized plasma, thus converting ISM material into a hot and very tenuous state. We shall see in this lecture that we cannot understand the large-scale dynamics of the ISM without profound knowledge of the underlying micro- physics. And vice versa, we will argue that dynamical processes on large galactic scales determine the local properties of the different phases of the ISM, such as their ability to cool and collapse, and to give birth to new stars. ISM dynamics spans a wide range of spatial scales, from the extent of the galaxy as a whole down to the local blobs of gas that collapse to form individual stars or binary systems. Similarly, it covers many decades in tem- poral scales, from the hundreds of millions of years it takes to complete one galactic rotation down to the hundreds of years it takes an ionization front to travel through a star-forming cloud. This wide range of scales is intricately Ralf Klessen & Simon Glover 5 linked by a number of competing feedback loops. Altogether, characterizing the ISM is truly a multi-scale and multi-physics problem. It requires insights from quantum physics and chemistry, as well as knowledge of magnetohy- drodynamics, plasma physics, and gravitational dynamics. It also demands a deep understanding of the coupling between matter and radiation, together with input from high-resolution multi-frequency and multi-messenger astro- nomical observations. By mass, the ISM consists of around 70% hydrogen (H), 28% helium (He), and 2% heavier elements. The latter are generally termed metals in the some- times very crude astronomical nomenclature. We give a detailed account of the composition of the ISM in Section2. Because helium is chemically inert, it is customary, and indeed highly practical, to distinguish the different phases of the ISM by the chemical state of hydrogen. Ionized bubbles are called Hii regions, while atomic gas is often termed Hi gas, in both cases referring to the spectroscopic notation. Hii regions are best observed by looking at hydrogen recombination lines or the fine structure lines of ionized heavy atoms. The properties of Hi gas are best studied via the 21cm hyperfine structure line of hydrogen. Dark clouds are sufficiently dense and well-shielded against the dissociating effects of interstellar ultraviolet radiation to allow H atoms to bind together to form molecular hydrogen (H2). They are therefore called molecular clouds. H2 is a homonuclear molecule. Its dipole moment vanishes and it radiates extremely weakly under normal Galactic ISM conditions. Direct detection of H2 is therefore generally possible only through ultraviolet absorption studies. Due to atmospheric opacity these studies can only be done from space, and are limited to pencil-beam measurements of the absorption of light from bright stars or active galactic nuclei (AGN). We note that rotational and ro- vibrational emission lines from H2 have indeed been detected in the infrared, both in the Milky Way and in other galaxies. However, this emission comes from gas that has been strongly heated by shocks or radiation, and it traces only a small fraction of the overall amount of molecular hydrogen. Due to these limitations, the most common tool for studying the molecular ISM is radio and sub-millimeter emission either from dust grains or from other molecules that tend to be found in the same locations as H2. By far the most commonly used molecular tracer is carbon monoxide with its various isotopologues. The most abundant, and hence easiest to observe is 12C16O, usually referred to simply as 12CO or just CO. However, this isotopologue is often so abundant that its emission is optically thick, meaning that it only traces conditions reliably in the surface layers of the dense substructure found within most molecular clouds. The next most abundant isotopologues are 13C16O (usually written simply as 13CO) and 12C18O (usually just C18O). Their emission is often optically thin and can freely escape the system. This allows us to
Details
-
File Typepdf
-
Upload Time-
-
Content LanguagesEnglish
-
Upload UserAnonymous/Not logged-in
-
File Pages191 Page
-
File Size-