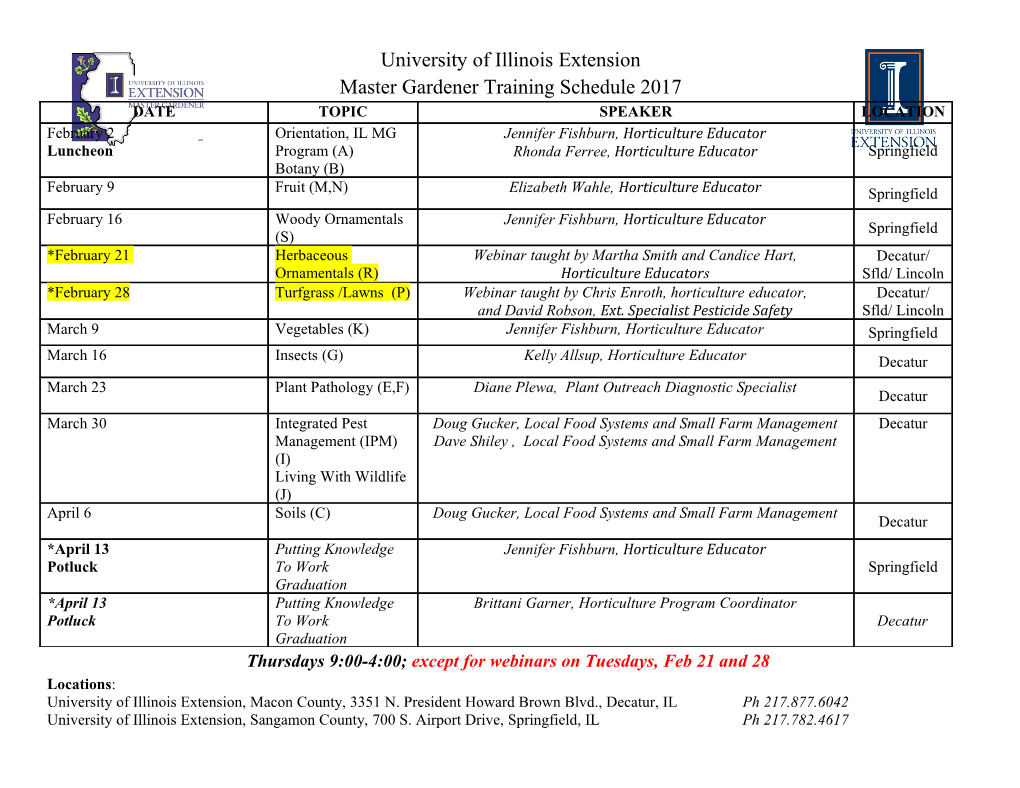
Can we predict the composition of an exoplanet? A Thesis Presented in Partial Fulfillment of the Requirements for the Degree Master of Science in the Graduate School of The Ohio State University By Joseph Gregory Schulze, B.S. Graduate Program in School of Earth Sciences The Ohio State University 2020 Master's Examination Committee: Dr. Wendy Panero, Advisor Dr. Joachim Moortgat Dr. Ji Wang c Copyright by Joseph Gregory Schulze 2020 Abstract Is the Earth unique in its habitability, or is it just one of many life-hosting planets in the universe? The key to answering this question lies in determining how rocky planets form and evolve, which can be inferred from how similar/dissimilar the com- positions of such planets are to their host stars. For instance, the terrestrial planets formed from a disk made of the same material as the Sun. The compositions of Earth, Venus, and Mars are consistent with the relative amounts of the major rock- building materials (Fe, Mg, Si) found in the Sun. Mercury, however, is much more iron-enriched relative to the Sun. This implies that it underwent a different forma- tion/evolutionary pathway, likely a single or series of catastrophic mantle-stripping collisions during the late stages of its formation. In short, Mercury and Earth have two very different histories, which is realized in how their compositions deviate from the Sun's. Therefore, understanding the relative importance of various formation and evolution processes for rocky exoplanets hinges on first determining how con- sistent the compositions of these planets are with their host stars. In this work, I develop a statistical framework for assessing the degree to which super-Earth's re- flect the refractory compositions of their host stars. I implement this framework on the 8 best-measured super-Earths for which the host star's composition is known and explore the possibility of secondary atmospheres to explain super-Earths with an apparent iron-depletion relative to their host. ii Acknowledgments I first want to thank my advisor, Dr. Wendy Panero. I am exceptionally grateful for the freedom Wendy has given me to explore the topics I find most exciting while also preventing me from going down endless rabbit holes. This thesis would not have been possible without her. I am excited to continue to grow as a scientist under her supervision as a Ph.D. student. I would also like to extend thanks to my committee members, Dr. Ji Wang and Dr. Joachim Moortgat, for their knowledge and insights provided during this research. I would also like to thank the members of the Panero Group and the Planet Group for their interest in my work. Working with these talented scientists has greatly expanded my research and exposed me to new ways of thinking about the same research questions. I wish to thank my parents, Tammy and Bob, and my siblings, Brad, Jen, Gabbert, and Millie for sitting through several practice talks and listening to my many exo- planet rambles. I would like to give a special shout-out to my Dad for first piquing my interest in exoplanets by giving me my first astronomy book, binge-watching and re-watching The Universe with me, and many, many late-night discussions. Thank you, Mr. Dad. Last, I want to thank my fellow SES graduate students for helping me navigate the waters of grad school and for providing a fun social outlet. I would especially like to thank Sam and her four-legged companion (aka The Beast) for their great presentation and writing suggestions, for finishing and restructuring my sentences, and for some much needed comedic relief during confinement. Now back to chorin'. iii Vita June 11, 1994 . Born - Columbus, OH, USA 2017 . B.S. Physics & Astronomy, The Ohio State University 2018-2019 . .University Fellow 2019-2020 . .Graduate Teaching Associate, The Ohio State University. Publications Research Publications Page, M. R., McCullian, B. A., Purser, C. M., Schulze, J. G., Nakatani, T. M., Wolfe, C. S., Childress, J. R., McConney, M. E., Howe, B. M., Hammel, P. C. and Bhallamudi,V. P., Optically detected ferromagnetic resonance in diverse ferromag- nets via nitrogen vacancy centers in diamond. Journal of Applied Physics, 126, 12. Fields of Study Major Field: Earth Sciences iv Table of Contents Page Abstract . ii Acknowledgments . iii Vita......................................... iv List of Tables . vii List of Figures . viii 1. Introduction . .1 2. Do the compositions of rocky exoplanets reflect their star's refractory abundances? . .9 2.1 Introduction . 10 2.2 Sample Selection . 11 2.3 Planetary Structure Calculations . 14 2.4 Hypothesis testing . 16 2.5 Results . 19 2.6 Discussion and Conclusions . 25 3. ExoLens { A Compositional Calculator for Rocky Worlds . 28 3.1 Introduction . 28 v 3.2 Analytical Expression for Core Mass Fraction as a Function of Plan- etary Observables, Mass and Radius . 29 3.3 Parameter Fitting . 31 3.4 Inputs and Outputs . 33 3.5 Comparison with Zeng et al. (2016a) . 37 4. A Simple Adiabatic Model for Secondary Atmospheres . 41 4.1 Introduction . 41 4.2 The physics of atmospheric escape . 42 4.3 Atmospheric Modeling . 45 4.4 Reproducing Earth and Venus' Atmospheres . 47 4.5 Discussion . 48 Appendices 53 A. Calculation of CMF and σCMF . 53 vi List of Tables Table Page 2.1 Selected sample of well-characterized exoplanets. M-R sources: (1) Dai et al. (2019), (2) Ligi et al. (2019), (3) Espinoza et al. (2019), (4) Bonomo et al. (2019). Spectroscopy sources: (1) Santerne et al. (2018). (2) Santos et al. (2015). (3) Hypatia Catalogue (median values) (Hinkel et al., 2014). (4) Hellier et al. (2012). (5) Espinoza et al. (2019). (6) Bonomo et al. (2019). 13 2.2 Inferred properties of the selected small, well-characterized exoplanets, classified as S.E., super-Earth, S.M. super-Mercury, or S.F. super- Fluff. P.R.C. = Previously Reported Class. Sources: (1) Santerne et al. (2018), (2) Brugger et al. (2017), (3) Dorn et al. (2019), (4) Espinoza et al. (2019). (5) Bonomo et al. (2019). 21 4.1 Initial Parameters for Earth and Venus from Earth Fact Sheet and Venus Fact Sheet, respectively. 52 4.2 Summary of Results. 52 A.1 The effects of Mp, Rp, σRp and σMp on CMFρ and σCMFρ ....... 54 A.2 The effects of Fe/Mg, Si/Mg, σFe/Mg and σSi/Mg on CMF? and σCMF? . 55 vii List of Figures Figure Page 2.1 Ternary diagrams of the CMF-MMF-WMF solution space for all plan- ets in our sample with known stellar Fe/Mg and Si/Mg ratios. All so- lutions are subject to the constraint that CMF+MMF+WMF = 100% 20 2.2 CMFρ and CMF? uncertainty maps as a function of observational uncertainties. (a) Uncertainty in CMFρ as a function of mass and radius uncertainties. We plot the values for Mp = 5:0M⊕ and Rp = 1:545R⊕, whereas values in Table 2.2 are exact. (b) Uncertainty in CMF? as a function of Fe/Mg and Si/Mg uncertainties. We use Fe/Mg = 0.98 and Si/Mg = 1.0. These choices give a central value of 0.35 for both CMFρ and CMF?. Uncertainties for additional planets with measured masses and radii without stellar Fe/Mg and Si/Mg are also included. Color labels are for clarity. 24 3.1 CMFρ vs. Rp curves for Mp = 0:01 (cyan) to 11M⊕ (magenta). Exo- Plex data points are denoted by squares. Fits to Equ. 3.9 are shown as solid lines. Fit residuals are shown in the top panel. While there is a clear systematic trend in the residuals, the amplitude of this trend is, at most, 0.02% which is 3 orders of magnitude smaller than the current uncertainties in CMFρ due to observational uncertainties in planetary mass and radius. 32 3.2 Fit to mean core density as a function of planetary mass. 34 3.3 Fit to mean mantle density as a function of planetary mass. 35 viii 3.4 Difference between CMFρ calculated from ExoPlex and ExoLens for 13 likely rocky exoplanets (Rp < 1:9R⊕) with σM < 20% and σR < 10%. R.M.S. = 1.4%. 36 3.5 Schematic overview of ExoLens. 38 3.6 Sample ExoLens output for K2-229b. Black solid lines correspond to nine equally-spaced confidence intervals between 10 and 90%. The dashed cyan lines are the 68% and 95% confidence intervals. (Bot- tom left) Contour lines (white) correspond to lines of constant CMFρ values. Negative values indicate M-R combinations that cannot be ex- plained by a purely MgSiO3 composition and require either an outer volatile layer or enrichment in ultra-refractory materials. (Bottom right) likelihood functions for CMFρ and CMF?............. 39 3.7 Comparison plot between Z16 and ExoLens. (Left) Z16 (dashed) and ExoLens (solid) M-R curves for varying core mass fractions. (Right) Difference in CMFρ between Z16 and ExoLens for Mp = 1:0 and 10:0M⊕. 40 4.1 Atmospheric particle mass where vthermal = vescape as a function of planet mass for 300, 1000, and 2000 K. Solid symbols for Earth, Venus, and 55 Cnc e are plotted according to their equilibrium temperature according to their orbital distance (no greenhouse). The open symbol for Venus reflects its present surface temperature. 46 4.2 My model of Earth's atmosphere (solid) compared with the 1976 U.S. standard atmosphere model (dashed). 49 4.3 My model of the Venetian atmosphere compared with Justus & Braun (2007) . 50 ix Chapter 1: Introduction The solar system contains 8 planets which can be broadly divided into two com- positional categories: the rock-dominated terrestrial planets and the light-element dominated gas/ice giants.
Details
-
File Typepdf
-
Upload Time-
-
Content LanguagesEnglish
-
Upload UserAnonymous/Not logged-in
-
File Pages70 Page
-
File Size-