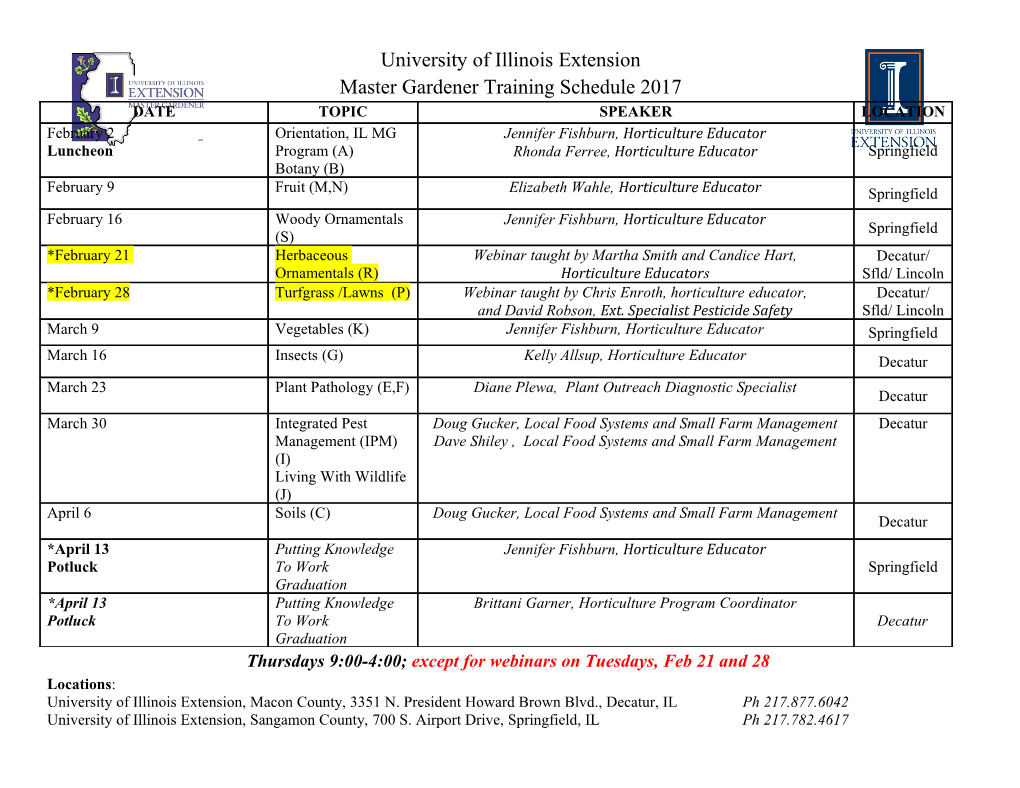
Specific Ion Effects on Hydrogen-Bond Rearrangements in the Halide–Dihydrate Complexes Pushp Bajaj,∗,y Debbie Zhuang,y and Francesco Paesani∗,y,z,{ yDepartment of Chemistry and Biochemistry, University of California, San Diego La Jolla, California 92093, United States zMaterials Science and Engineering, University of California, San Diego La Jolla, California 92093, United States {San Diego Supercomputer Center, University of California, San Diego La Jolla, California 92093, United States E-mail: [email protected]; [email protected] 1 Abstract Small aqueous ionic clusters represent ideal systems to investigate the microscopic hydrogen- bonding structure and dynamics in ion hydration shells. In this context, halide-dihydrate com- plexes are the smallest systems where the interplay between halide–water and water–water interactions can be studied simultaneously. Here, quantum molecular dynamics simulations − unravel specific ion effects on the temperature-dependent structural transition in X (H2O)2 complexes (X = Cl, Br and I) which is induced by the breaking of the water–water hydrogen bond. A systematic analysis of the hydrogen-bonding rearrangements at low temperature pro- vides fundamental insights into the competition between halide–water and water–water inter- actions depending on the properties of the halide ion. While the halide–water hydrogen-bond − − strength decreases going from Cl (H2O)2 to I (H2O)2, the opposite trend in observed in the strength of the water–water hydrogen-bond, suggesting that non-trivial many-body effects may also be at play in the hydration shells of halide ions in solution, especially in frustrated systems (e.g., interfaces) where the water molecules can have dangling OH bonds. Graphical TOC Entry Ground State Tunneling Splitting - - - Cl (H2O)2 Br (H2O)2 I (H2O)2 Halide-Water H-bond Bifurcation Water-Water H-bond Bifurcation Rotation Flip 2 Ionic aqueous systems are ubiquitous. For example, they occur inside living cells1–6 and in the environment,7–9 and are central components of many devices, including electrolytic cells, capaci- tors, and batteries.10 An accurate characterization of the underlying interactions between the ions and the surrounding water molecules is central for understanding specific ion effects as well as for controlling the underlying physicochemical processes. While there have been extensive exper- imental and computational studies on ion hydration,8,11–17 a definitive picture of how the ions are hydrated and to what extent structural differences in the organization of the hydration shells trans- late into differences in the thermodynamic and dynamical properties of the corresponding ionic systems is still lacking. Ion-water clusters are ideal systems for characterizing the molecular mechanisms associated with hydrogen-bonding (H-bonding) rearrangements within the hydration shells of an ion, since, due to their relatively small sizes, they are amenable to high-level molecular modeling12,18–36 and, at the same time, can be studied experimentally using high-resolution vibrational spectroscopy.37–40 In this context, halide dihydrates hold a special place since they are the smallest complexes in which two water molecules can be simultaneously H-bonded to each other and to the ion, thus allowing for directly probing the interplay between ion–water and water–water interactions. Building upon our recent study of isotope effects and tunneling in the isotopomers of the − 34 I (H2O)2 complex, we report here a systematic analysis of the structure and temperature de- − pendent evolution of the X (H2O)2 dihydrates (with X = Cl, Br and I), which provides funda- mental insights into how halide ions with different size, charge density and polarizability affect the H-bond rearrangement dynamics within similar complexes. Anharmonic vibrational frequency calculations and path integral molecular dynamics (PIMD) simulations performed for all three halide–dihydrates using the halide-water MB-nrg many-body potential energy functions (PEFs) introduced in Refs. 32 and 33 predict a transition from closed cyclic arrangements, where the two water molecules are H-bonded to the halide ion and to each other, to open arrangements, where the water–water H-bond is broken. At low temperature, when the complexes exist predominantly in the closed configuration, ring-polymer instanton (RPI) calculations provide unambiguous evi- 3 dence for H-bond rearrangements taking place in all three halide–dihydrates via quantum tunneling mediated by the presence of the halide ion. To provide context for our analysis, anharmonic vibrational frequencies calculated for the − global minimum energy configurations of all three X (H2O)2 dihydrates (Fig. 1a) using the com- bined local-mode41,42 and local-monomer43 methods (see Supporting Information for details) are compared in Fig. 1b with the corresponding values measured in argon tagged-vibrational predisso- 23,38 − ciation experiments. The minimum energy configuration of each X (H2O)2 complex exhibits a cyclic arrangement where the two water molecules form H-bonds to the halide ion and a single H-bond to each other. Based on the H-bonding environment within the complex, the two water molecules are classified as donor-donor (DD), donating two H-bonds, and acceptor-donor (AD), accepting one H-bond and donating one H-bond, respectively. As a consequence, the four OH bonds, labelled as IHBDD, IHBAD, IH and FOH, are associated with four distinct vibrational fre- − quencies (Fig. 1). For all three X (H2O)2 complexes, the calculated anharmonic frequencies are in excellent agreement with the corresponding experimental values, providing further evidence 32,33 for the accuracy of the MB-nrg PEFs. Clearly, the IHBDD and IHBAD vibrational frequencies, corresponding to the two H-bonded-to-halide positions, increase going from chloride to iodide as a) b) FOH IH IHBAD IHB DD Figure 1: a) Schematic representation of the global minimum energy configuration of the three halide–dihydrate complexes analyzed in this study. b) OH-stretch vibrational frequencies (in −1 − − − cm ) for the Cl (H2O)2, Br (H2O)2 and I (H2O)2 complexes. Experimental values for the − − − Cl (H2O)2 and Br (H2O)2 clusters are taken from Ref. 23 and while those for the I (H2O)2 com- plex are taken from Ref. 38. 4 the halide–water H-bond strength decreases. On the other hand, there is a slight decrease in the IH vibrational frequency corresponding to the H-bonded-to-water position, suggesting an increase − − in the water–water H-bond strength going from Cl (H2O)2 to I (H2O)2. These findings are in agreement with previous studies assessing the trends in the halide–water and water-water interac- tions in small halide–water clusters which suggest that the presence of the halide ion weakens the water–water H-bond strength.12,23–25,44 − The variation of the water–water H-bond strength in the X (H2O)2 complexes can be further quantified through the analysis of one-dimensional potential energy scans along the O-X-O0 angle (denoted as β in Fig. 2a) characterizing the transition from closed to open configurations. As shown in Fig. 2b, each of the three potential energy scans exhibits a minimum value for β corresponding to the closed configuration with an intact water–water H-bond and then plateaus for larger values of β as the water–water H-bond breaks. The energy required to break the water–water H-bond a) b) c) d) e) f) Figure 2: a) Schematic representation of the O-X-O0 (β) angle used to classify closed and open configurations. b) One dimensional potential energy scans along the β angle. c) Relative popu- lations of the closed configurations calculated from PIMD simulations carried out as a function − − − of temperature for the Cl (H2O)2, Br (H2O)2 and I (H2O)2 complexes. d)–f) Normalized prob- ability distributions of the β angle calculated from PIMD simulations carried out as a function of − − − temperature for the Cl (H2O)2, Br (H2O)2 and I (H2O)2 complexes, respectively. 5 − is the smallest for the Cl (H2O)2 complex which, consequently, is characterized by the weakest water–water H-bond among the three halide–dihydrates. In order to characterize the structural evolution of all three complexes as a function of tem- perature, normalized probability distributions of the β angle computed from PIMD simulations are − − − shown in Fig. 2d, 2e and 2f for Cl (H2O)2, Br (H2O)2 and I (H2O)2, respectively. At low temper- ature, all three distributions are spread over narrow ranges of β, where the complexes exist exclu- sively in closed configurations. As the temperature increases, a shoulder starts to appear for larger values of β, indicating that the water–water H-bond start breaking and the complex transitions to the open configuration. This transition can be easily quantified by classifying the instantaneous molecular configurations as closed or open using a cutoff value for β and calculating the relative populations of both closed and open configurations as a function of temperature (Fig. 2c. A cutoff ◦ ◦ ◦ ◦ ◦ ◦ − − value of β = 70 ± 2 , 64 ± 2 , and 60 ± 2 was chosen for the Cl (H2O)2, Br (H2O)2 and − I (H2O)2 complexes, respectively, to classify the configurations as closed. Since the water–water − − H-bond strength increases going from Cl (H2O)2 to I (H2O)2, the water–water H-bond starts to break at lower temperature (∼75 K) in the chloride–dihydrate complex and at higher temperature (∼100 K) in both bromide– and iodide–dihydrate complexes. As entropic contributions become dominant at higher temperature, the small energy differences between the three halide–dihydrates are diminished and the corresponding distributions overlap. While the large-amplitude fluctuations leading to breaking of the water–water H-bond can be probed through experimental vibrational spectroscopy, the faster water rotational motions leading to H-bond rearrangements within each complex cannot be detected using current experimental se- tups.
Details
-
File Typepdf
-
Upload Time-
-
Content LanguagesEnglish
-
Upload UserAnonymous/Not logged-in
-
File Pages17 Page
-
File Size-