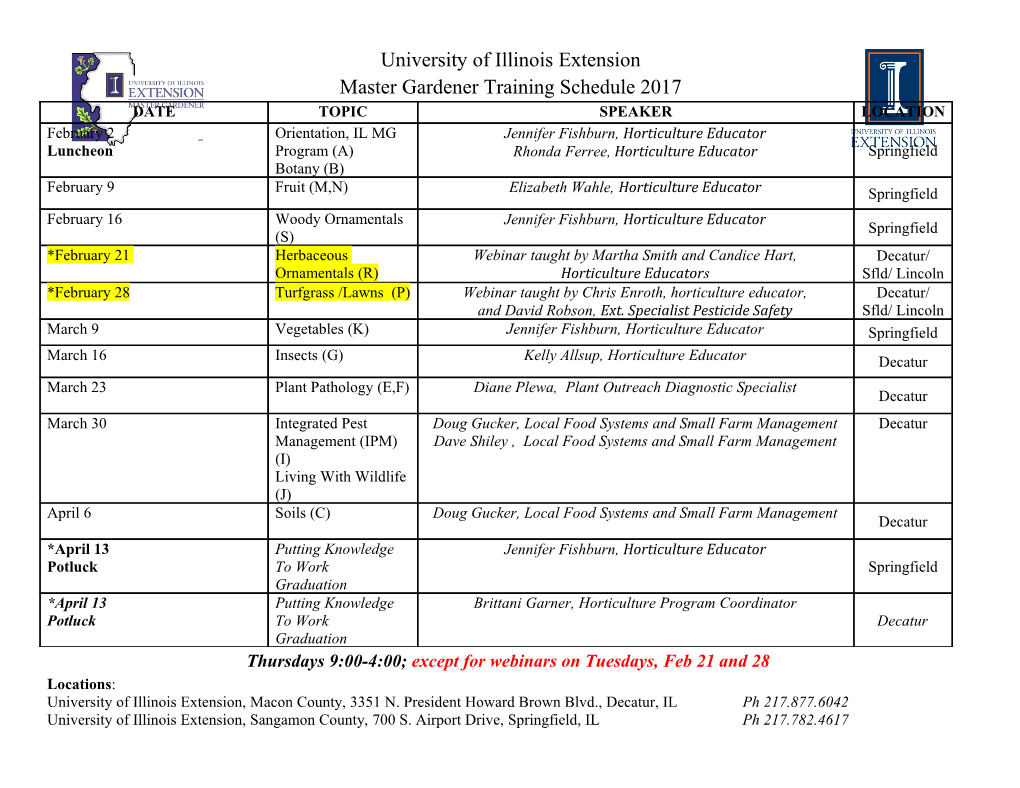
Lie Algebras Tudor Ciurca January 19, 2020 Contents 1 Course information 2 2 Introduction 3 2.1 Engel's theorem . .6 2.2 Lie's theorem . .8 3 Classification 10 1 1 Course information Office Hour: Mondays 12:00 - 13:00 Coursework: 2 tests and 1 assessed coursework All purple text was added post-lecture. 2 2 Introduction Definition 2.1. A Lie algebra a over a field K is a K-vector space quipped with a bilinear product [; ] satisfying 1. [a; a] = 0 for all a 2 a 2. [a; [b; c]] + [b; [c; a]] + [c; [a; b]] = 0 for all a; b; c 2 a Definition 2.2. A Lie algebra a is called Abelian if [a; b] = 0 for all a; b 2 a. Proposition 2.3. Let a be a Lie algebra. Then [; ] is skew-symmetric so that [a; b] = −[b; a] for all a; b 2 a. Proof. The result follows directly from 0 = [a + b; a + b] = [a; a] + [b; b] + [a; b] + [b; a] = [a; b] + [b; a] Definition 2.4. A morphism of Lie algebras f : a ! b is a K-linear map satisfying [f(x); f(y)] = f([x; y]) for all x; y 2 a. Proposition 2.5. There is a functor Θ: AlgK ! LieK for any field K that sends any K-algebra A to its associated Lie algebra Θ(A) with Lie bracket defined by [a; b] = ab − ba for all a; b 2 A.A K-algebra map becomes a morphism of Lie algebras. Proof. One firstly verifies that Θ(A) is indeed a Lie algebra 1.[ a; a] = a2 − a2 = 0 for all a 2 A 2.[ a; [b; c]] + [b; [c; a]] + [c; [a; b]] = a[b; c] − [b; c]a + b[c; a] − [c; a]b + c[a; b] − [a; b]c = abc − acb − bca + cba + bca − bac − cab + acb + cab − cba − abc + bac = 0 for all a; b; c 2 A. Suppose f : A ! B is a map of K-algebras. Then it is clearly K-linear, and f([a; b]) = f(ab − ba) = f(ab) − f(ba) = f(a)f(b) − f(b)f(a) = [f(a); f(b)] Thus f becomes a morphism of Lie algebras. Example 2.6. 1. The previous proposition gives us a lot of examples. Notable examples include gln(K) := Θ(Gln(K)) for a field K. Many examples are not of this form, but we can get many more as subalgebras of such Lie algebras, as you can see below. 2. sln(K) ⊂ gln(K) defined as the subspace of zero trace matrices. Closure under Lie bracket is easily verified since T r(ab) = T r(ba) for all matrices a; b 2 gln(K). T 3. son(K) ⊂ gln(K) defined as the subspace of skew-symmetric matrices. Verify that (ab−ba) = bT aT − aT bT = ba − ab = −(ab − ba) 4. tn(K) ⊂ gln(K) defined as the subspace of upper triangular matrices. This is in fact a subring so clearly it is also a Lie subalgebra with the inherited Lie bracket. 3 Definition 2.7. Let a be a Lie algebra over K. A Lie subalgebra is a subspace b ⊂ a closed under the Lie bracket of a. Clearly b becomes a Lie algebra. An ideal in a is a Lie subalgebra b ⊂ a satisfying [a; b] 2 b for all b 2 b and a 2 a. The quotient Lie algebra a=b is the corresponding quotient vector space with Lie bracket inherited from a. To prove this is well defined, suppose a − b 2 b and x − y 2 b for some a; b; x; y 2 a. Then [a; x] − [b; y] ≡ [a; x] − [b; y] + [b; y − x] + [b − a; x] ≡ 0 (mod b) Remark 2.8. The kernel of a Lie algebra morphism is an ideal. Let φ : a ! b be a Lie algebra morphism, and consider the subspace ker(φ) ⊂ a. We verify that for all x 2 ker(φ) and y 2 a we have φ[x; y] = [φ(x); φ(y)] = [0; φ(y)] = 0 and so [x; y] 2 ker(φ) as required. Definition 2.9. Let a be a Lie algebra, with ideals m; n. We define the Lie subalgebra [m; n] to be the subspace of a spanned by the set f[m; n]: m 2 m; n 2 ng. This inherits the Lie bracket of a. It becomes an ideal of a since for all x 2 m,a 2 n and t 2 a. [t; [x; a]] = −[x; [a; t]] − [a; [t; x]] = [n; x] + [m; a] 2 [m; n] for n = [a; t] 2 n and m = [t; x] 2 m. Definition 2.10. Let a be a Lie algebra. We define the derived series of a via the following • a(0) = a • a(n+1) = [a(n); a(n)] (n) for all n 2 N. Each a is an ideal of a by inductively applying Definition 2.9. (n) Definition 2.11. A Lie algebra a is said to be solvable if a = 0 for some n 2 N. Every abelian Lie algebra is solvable. Proposition 2.12. Let a be a Lie algebra and [a; a] its derived subalgebra. Then the Lie algebra a0 = a=[a; a] is Abelian. Proof. For all x; y 2 a0 we have [x; y] ≡ 0 by definition and so we are done. Definition 2.13. Let a be a Lie algebra. We define the (lower) central series series of a via the following • a0 = a • an+1 = [an; a] n for all n 2 N. Each a is an ideal of a by inductively applying Definition 2.9. n Definition 2.14. A Lie algebra a is said to be nilpotent if a = 0 for some n 2 N. Every abelian Lie algebra is nilpotent, and every nilpotent Lie algebra is solvable. This is clear since a0 = a(0) and if we assume by induction that an ⊃ a(n) then an+1 = [an; a] ⊃ [a(n); a(n)] = a(n+1). Definition 2.15. Let a be a Lie algebra over a field K. A representation of a is a Lie algebra morphism φ : a ! gl(V ) for some vector space V over K. Example 2.16. We have the adjoint representation ad : a ! gl(a) defined by ad(x) = [x; ]. 4 Proof. ad is K-linear since ad(kx + y) = [kx + y; ] = k[x; ] + [y; ] for all k 2 K and x; y 2 a, by bilinearity of the Lie bracket. Now for all x; y; z 2 a we show ad([x; y])(z) = [[x; y]; z] = −[z; [x; y]] = [x; [y; z]] + [y; [z; x]] = = [x; [y; z] − [y; [x; z]] = ([x; ][y; ] − [y; ][x; ])(z) = [ad(x); ad(y)](z) Definition 2.17. The center of a Lie algebra a over a K is ker(ad) = fz 2 a :[z; x] = 0 8x 2 ag Therefore the adjoint representation induces an injective (or faithful) representation of the Lie algebra a=ker(ad) into gl(a). The adjoint representation will be of great use to us. Definition 2.18. Suppose φ : a ! gl(V ) is a representation of a. Let W ⊂ V be a K-subspace which is φ(a)−invariant so that φ(x)(w) 2 w for all w 2 W and x 2 a. Then we get an induced quotient representation φW : a ! gl(V=W ). We will also be using this construction often. It is easy to verify that we do indeed get a mor- phism of Lie algebras φW : a ! gl(V=W ). Proposition 2.19. Let b ⊂ a be a Lie subalgebra. Then the following are true 1. If a is solvable, then b is solvable. 2. If a is nilpotent, then b is nilpotent. Proof. It suffices to prove by induction that b(n) ⊂ a(n) and bn ⊂ an. The base case is precisely the assumption b ⊂ a. Suppose that bn−1 ⊂ an−1 and b(n−1) ⊂ a(n−1). Then bn = [bn−1; b] ⊂ [an−1; a] = an and b(n) = [b(n−1); b(n−1)] ⊂ [a(n−1); a(n−1)] = an so we are done. We may refine this result when we are dealing with ideals in the solvable case. Proposition 2.20. Let a be a Lie algebra with an ideal b ⊂ a. Then a is solvable if and only if b and a=b are solvable. Proof. The forward direction is clear. Let us assume that b and a=b are solvable. Then there (n) is some n 2 N so that a ⊂ b under the assumption that a=b is solvable. Now there is also (m) some m 2 N so that b = 0 under the assumption that b is solvable. Finally we see that a(m+n) = (a(n))(m) = b(m) = 0. Proposition 2.21. Suppose a is a Lie algebra with an ideal b ⊂ a. Then there is a one-to- one inclusion preserving correspondence between ideals containing b and ideals of the quotient Lie algebra a=b. Proof. An ideal of a containing b naturally yields an ideal of a=b through the quotient. Now take an ideal c ⊂ a=b and define c0 = b + c as a vector space. Then c0 is an ideal since given b 2 b, c 2 c and a 2 a we have [a; b + c] = [a; b] + [a; c] 2 c0 since [a; b] 2 b and [a; c] 2 c. One can now easily check that this correspondence is inclusion preserving.
Details
-
File Typepdf
-
Upload Time-
-
Content LanguagesEnglish
-
Upload UserAnonymous/Not logged-in
-
File Pages10 Page
-
File Size-