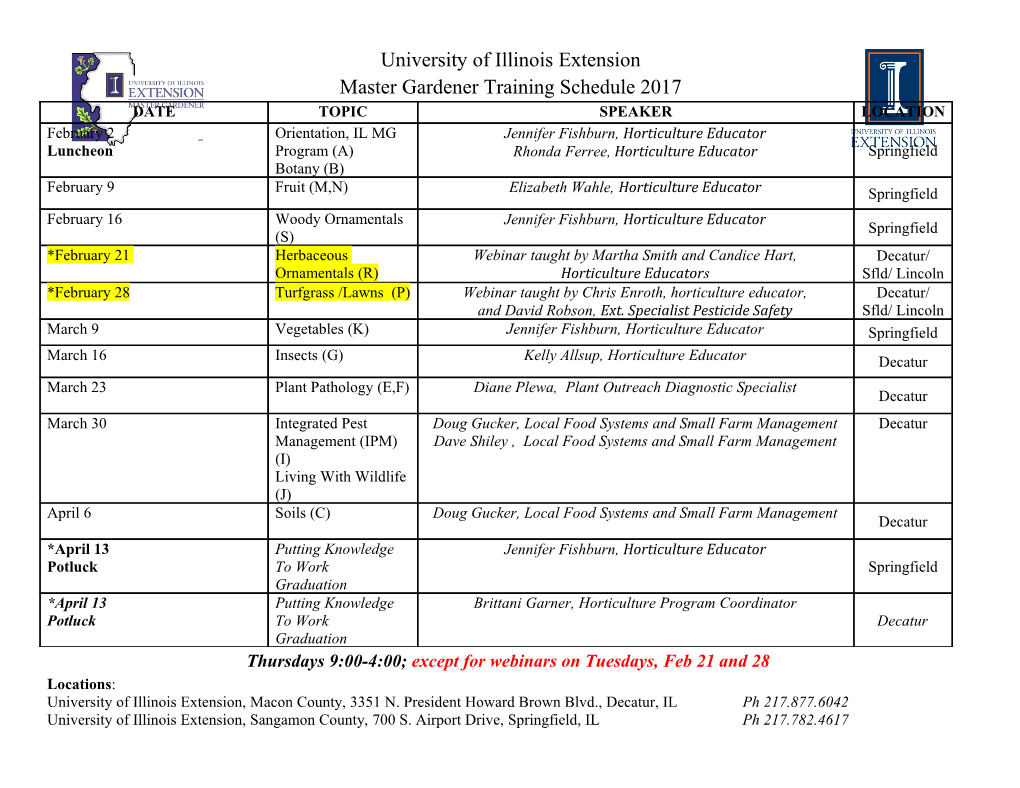
Using Saccharomyces cerevisiae to characterise the in vivo effects of exposure to the prion-curing drug Tacrine and the fungal metabolite gliotoxin Jennifer O’Brien B.Sc. A thesis submitted to the National University of Ireland for the degree of Doctor of Philosophy May 2012 Supervisor Head of Department Dr. Gary Jones Prof. Kay Ohlendieck Department of Biology Department of Biology National University of Ireland National University of Ireland Maynooth Maynooth Co. Kildare Co. Kildare Acknowledgements Firstly, I would like to sincerely thank Dr. Gary Jones for giving me the opportunity to work in the Yeast Genetics lab and for his support, guidance and advice over the last three years. I would like to thank Professor Marc Blondel and Dr. Cecile Voisset for reagents and strains and Dr. David Fitzpatrick for help with bioinformatics analysis. Thanks to Grainne and the girls from Muscle Biology, Lisa and Edel who helped me with protein work and to Eoin for his patience when I bombarded him with qPCR questions. I feel so lucky to have worked with great people during my PhD and to have made such good friends. Thanks to Ciara, Sarah and Emma for showing me the ropes, answering my constant questions and being such good craic, you girls made settling in so easy! Ste and Naushie, you two did a brilliant job taking over, really enjoyed working and drinking coffee with you, keep up the good work! I want to thank members of the Biotechnology and Medical Mycology labs for advice on experiments and laughs in the coffee room. A special thanks to Niamhio for being a great friend over the years, inside and outside college. I would like to thank my three best friends Amanda, Fi and Ash for their constant encouragement even though they regularly didn’t really have a clue what I was talking about. A massive thanks to my three parents and Dave for supporting me through this process, for being professionals at feigning interest and saying all the right things. I really appreciate everything you have done for me. Nuno, I could not have done this without you, thank you so much for being patient, supportive and encouraging and for being the first person there when I needed to moan or rant! Declaration of Authorship This thesis has not previously been submitted in part to this or any other university and is the sole work of the author. ___________________________ Jennifer O’Brien, B.Sc. List of abbreviations AB Ammonium bicarbonate ABD ATPase-binding domain ABPA Allergic bronchopulmonary aspergillosis ACh Acetylcholine AChR Acetylcholine receptor ACN Acetonitrile AdoMet S-adenosyl-methionine A. fumigatus Aspergillus fumigatus Amp Ampicillin 6AP 6-aminophenanthridine APP Amyloid precursor proteins APS Ammonium persulfate ARE AP-1 recognition element BSA Bovine serum albumin BSE Bovine spongiform encephalopathy [14 C] Carbon-14 CJD Creutzfeldt–Jakob disease CWD Chronic wasting disease CuZnSOD Copper- and zinc-containing SOD dH 2O Distilled water Da Dalton DTT Dithiothreitol EB Elution buffer E. coli Escherichia coli EDTA Ethylenediaminetetraacetic acid eRF Eukaryotic release factor ETP Epipolythiodioxopiperazine FSE Feline spongiform encephalopathy FeSOD Iron-containing SOD GA Guanabenz GdnHCl Guanidine Hydrochloride GFP Green fluorescent protein Grx Glutaredoxin GSH Reduced glutathione GSS Gerstmann–Straussler–Sheinker disease GSSG Oxidised glutathione GT Gliotoxin HPLC High-performance liquid chromatography Hr Hour(s) HSP Heatshock protein H2O Water H2O2 Hydrogen peroxide IA Invasive aspergillosis IEF Isoelectric focusing kDA Kilodalton L Litre LB Luria broth LC-MS Liquid Chromatography Mass Spectrometry M Molar mA Milliamps Min Minute(s) ml Millilitre mM Millimolar MnSOD Manganese-containing SOD NEF Nucleotide exchange factor NiSOD Nickel-containing SOD NRPS Nonribosomal peptide synthetase OD Optical density OH Hydroxyl radical OS Oxidative stress - O2 Superoxide anion PBD Peptide-binding domain PCR Polymerase chain reaction PEG Polyethylene glycol PMSF Phenylmethylsulfonyl fluoride PrD Prion domain PSB Protein sample buffer RFM Ribosomal folding modulators ROS Reactive oxygen species RPFA Ribosome-borne protein folding activity rpm Repetitions per minute RT Room temperature S. cerevisiae Saccharomyces cerevisiae SC Synthetic complete SDS-PAGE Sodium Dodecyl Sulphate Polyacrylamide gel electrophoresis Sec Second(s) SOD Superoxide dismutase TA Tacrine TBS Tris buffered saline TSE Transmissible spongiform encephalopathy Trx Thioredoxin TrxR Thioredoxin reductase YNB Yeast nitrogen base YPD Yeast peptone dextrose YPGAL Yeast peptone galactose V Volts v/v volume/volume w/v weight/volume µm Micrometer µl Microlitre List of figures Figure 1.1 Images of A. fumigatus , A. fumigatus biofilm on bronchial epithelial cells, aspergilloma in lung of child suffering from leukaemia. Figure 1.2 Structure of gliotoxin. Figure 1.3 12-gene gliotoxin biosynthetic cluster, gli cluster. Figure 1.4 Redox cycling of GT within the cell induces ROS production and oxidative stress. Figure 1.5 Comedic advertisement warning against the contraction of Kuru which can be transmitted through brain consumption, sponge-like legion in the brain tissue of a CJD patient, sheep displaying weight loss and behaviour characteristic of scrapie infection. Figure 1.6 Structure of the S. cerevisiae Sup35p. Figure 1.7 Illustration of aggregated yeast PrD fused to GFP to enable detection in a [ PSI +] strain. Figure 1.8 Proposed model illustrating the importance of Hsp70, co-chaperones and NEFs in prion propagation and maintenance within the cell. Figure 1.9 Representation of the importance of chaperone activity cooperation in prion propagation. Figure 1.10 Structure of 6AP. Figure 1.11 Plate assay showing the effects of different compounds on [ PSI +] cells. Figure 1.12 Representation of 6AP anti-prion activity. Figure 1.13 Structure of GA. Figure 1.14 Structure of TA. Figure 2.1 Monitoring the presence/absence of [ PSI +] using a simple colour assay. Figure 2.2 Consequences of [ PSI +]. Figure 2.3 Cloning GliT into pC210. Figure 2.4 Method used to knock out genomic HBN1 in BY4741 with HIS5 . Figure 2.5 Method used to knock out genomic HNB1 in BY4741 ∆frm2 with HIS5 . Figure 2.6 Example of how qPCR 96-well plate may be laid out. Figure 3.1 Glutathione biosynthesis pathway in yeast. Figure 3.2 Gliotoxin inhibits the growth of S. cerevisiae . Figure 3.3 Gliotoxin suppresses yeast growth. Figure 3.4 Inhibitory effect of 16 and 64 µg/ml gliotoxin on BY4741 growth. Figure 3.5 Growth of wild-type strains in liquid culture containing gliotoxin. Figure 3.6 The ability of BY4741 to grow after gliotoxin exposure in liquid culture. Figure 3.7 Comparative growth analysis of BY4741 and ∆cys3 . Figure 3.8 Comparative growth analysis of BY4741, ∆yap1 and ∆sod1 . Figure 3.9 PCR amplified GSH1 . Figure 3.10 Plasmid maps constructed for pRS315 and GSH1 -pRS315. Figure 3.11 Comparative growth analysis of BY4741, ∆gsh1 and ∆gsh1 GSH1 -pRS315. Figure 3.12 Comparative growth analysis of BY4741, ∆ctt1 , ∆ace2 , ∆gsh2 , ∆glr1 and ∆trx2 under gliotoxin exposure. Figure 3.13 Comparative growth analsysis of BY4741, ∆gsh1 , ∆yap1 and ∆sod1 in response to various concentrations of H 2O2. Figure 3.14 Genomic sequence of GliT . Figure 3.15 Plasmid maps constructed for pC210 and GliT -pC210. Figure 3.16 Agarose gel illustrating GliT cloned into pC210 vector. Figure 3.17 Agowa sequencing result. Figure 3.18 Comparative growth analysis of BY4741 and ∆cys3 under gliotoxin exposure. Figure 3.19 Single colony growth assay of BY4741 under gliotoxin exposure. Figure 3.20 Coomassie stain of SDS-PAGE gel with protein from strains containing GliT -pC210. 1 = BY4741 GliT -pC210; 2 = BY4741 pRS315; 3 = G600 GliT -pC210; 4 = G600 pRS315; 5 = BY4741 ∆cys3 GliT -pC210; 6 = BY4741 ∆cys3 pRS315. Figure 3.21 NADPH oxidase activity in BY4741 when GliT is present. Figure 3.22 NADPH oxidase activity in BY4741 and G600 ( GliT -pC210). Figure 3.23 Plasmid maps constructed for pYES2 and GliT -pYES2. Figure 3.24 Diagnostic PCR depicting the cloning of GliT into pYES2. Figure 3.25 Comparative growth analysis of wild-type strains during metabolism of glucose and galactose (48 hr 30ºC). Figure 3.26 Comparative growth analysis of wild-type strains during metabolism of glucose and galactose with 8 µg/ml gliotoxin addition (48 hr 30ºC). Figure 3.27 Comparative growth analysis of BY4741 during galactose metabolism with 8 µg/ml gliotoxin addition (48 hr 30ºC + 48 hr RT). Figure 3.28 Coomassie stain of SDS-PAGE gel with protein from BY4741 containing GliT -pYES2 and pYES2 (chronic and acute galactose metabolism) 1 = GliT -pYES2 (acute); 2 = pYES2 (acute); 3 = GliT - pYES2 (chronic); 4 = pYES2 (chronic). Figure 3.29 NADPH oxidase activity in BY4741 ( GliT -pYES2). Figure 3.30 The percentage of each cellular component category (16 µg/ml upregulated genes). Figure 3.31 The percentage of each molecular function category (16 µg/ml upregulated genes). Figure 3.32 The percentage of each biological process category (16 µg/ml upregulated genes). Figure 3.33 The five associated cellular components most highly upregulated by exposure to 16 µg/ml gliotoxin. Figure 3.34 The five molecular functions most highly upregulated by exposure to 16 µg/ml gliotoxin. Figure 3.35 The five biological processes most highly upregulated by exposure To 16 µg/ml gliotoxin. Figure 3.36 The percentage of each cellular component category (16 µg/ml downregulated genes). Figure 3.37 The percentage of each molecular function category (16 µg/ml downregulated genes). Figure 3.38 The percentage of each biological process category (16 µg/ml downregulated genes). Figure 3.39 The five associated cellular components most highly downregulated by exposure to 16 µg/ml gliotoxin. Figure 3.40 The five molecular functions most highly downregulated by exposure to 16 µg/ml gliotoxin. Figure 3.41 The five biological processes most highly downregulated by exposure to 16 µg/ml gliotoxin. Figure 3.42 The percentage of each cellular component category (64 µg/ml upregulated genes).
Details
-
File Typepdf
-
Upload Time-
-
Content LanguagesEnglish
-
Upload UserAnonymous/Not logged-in
-
File Pages440 Page
-
File Size-