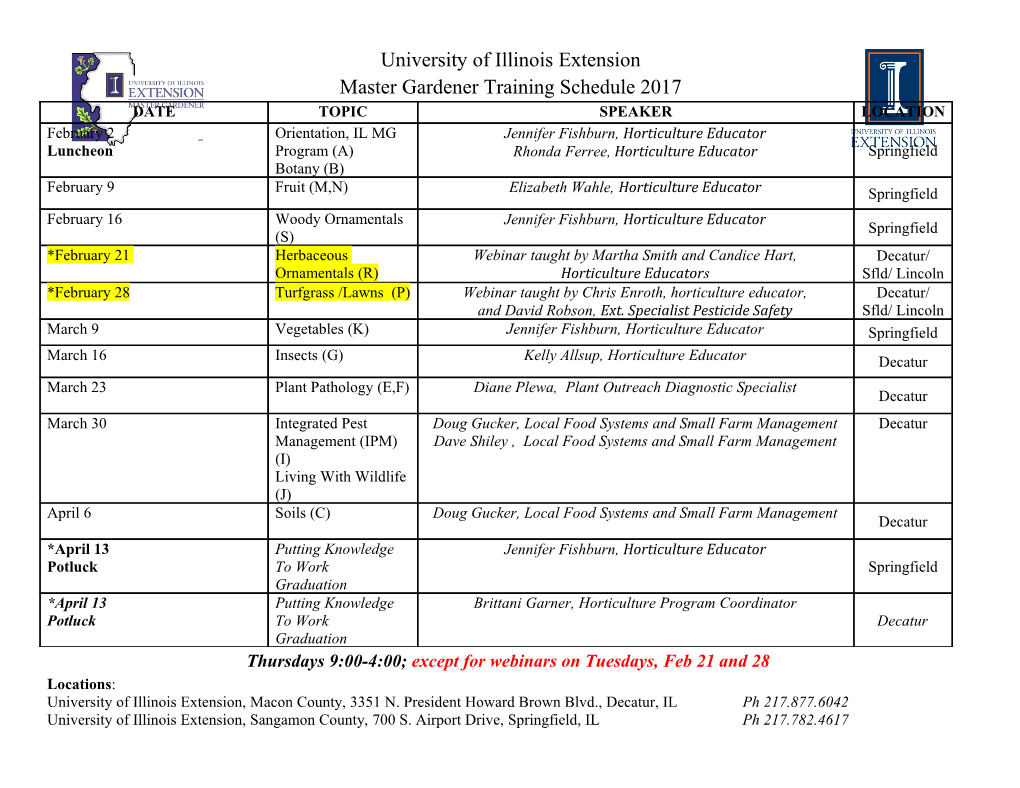
Dark matter lecture Teresa Marrod´anUndagoitia Max-Planck-Institut f¨urKernphysik, Saupfercheckweg 1, 69117 Heidelberg, Germany E-mail: [email protected] Abstract. This document is a brief summary of the topics discussed during the experimental part of the the dark matter lecture held together with Tilman Plehn in the winter term of 2015 at the University of Heidelberg. CONTENTS Contents 1 Dark matter indications4 1.1 Dispersion velocities of galaxies in clusters.................4 1.2 Rotation curves................................4 1.3 Gravitational lensing.............................4 1.4 Galaxy-cluster collisions...........................6 1.5 Cosmic microwave background........................7 1.6 Large structure formation..........................9 1.7 Big Bang nucleosynthesis........................... 10 2 Explanations and particle candidates 12 2.1 Explanations................................. 12 2.1.1 Modification of gravitational laws.................. 12 2.1.2 MACHOS and micro-lensing measurements............. 13 2.2 Particle properties to be the dark matter.................. 13 2.3 Standard model particles........................... 13 2.4 Beyond the standard model......................... 13 2.5 WIMPs and their production mechanism.................. 14 2.6 Supersymmetry (SUSY)........................... 15 2.7 Extra dimensions............................... 15 2.8 Superheavy WIMPs.............................. 16 2.9 Sterile neutrinos................................ 16 2.10 Axions..................................... 17 3 Dark matter distribution 19 3.1 Motivation................................... 19 3.2 Objects of interest.............................. 19 3.2.1 Structure of the Milky Way..................... 19 3.2.2 Milky Way satellite galaxies (dwarf spheroidals).......... 20 3.2.3 Other objects............................. 20 3.3 Simulations of dark matter halos...................... 21 3.3.1 Historical developments....................... 21 3.3.2 Density profile and substructure................... 22 3.3.3 Velocity distribution......................... 23 3.4 Uncertainties in indirect detection...................... 23 3.5 The standard halo model (SHM)...................... 24 3.6 Uncertainties in the determination of halo parameters........... 24 4 Indirect detection 25 4.1 Locations of indirect signals......................... 25 4.2 Possible processes and signal rates...................... 25 4.3 Particle propagation and detection..................... 25 2 CONTENTS 4.4 Searches using neutrinos........................... 25 4.5 Gamma detection in Cherenkov telescopes................. 28 4.6 Gamma detection by satellites........................ 31 4.7 X-rays: XMM-Newton & Chandra signal and interpretations....... 33 4.8 Charged particles............................... 35 5 Direct detection 37 5.1 Principles................................... 37 5.2 Backgrounds.................................. 43 5.2.1 External backgrounds: gammas, neutrons and neutrinos..... 43 5.2.2 Internal and surface backgrounds.................. 46 5.3 Statistical treatment of data......................... 47 5.4 Detector calibration.............................. 48 5.5 Experiments and results........................... 51 5.5.1 Scintillator crystals.......................... 51 5.5.2 Germanium detectors......................... 52 5.5.3 Cryogenic bolometers......................... 52 5.5.4 Liquid noble-gas detectors...................... 52 5.5.5 Superheated fluids.......................... 52 5.5.6 Directional searches.......................... 53 6 Dark matter searches at particle accelerators 53 6.1 Introduction.................................. 53 6.2 Signatures and features............................ 53 6.3 Searches at LEP............................... 53 6.4 Searches at LHC............................... 53 6.5 Comparison to direct/indirect detection results............... 53 3 1 DARK MATTER INDICATIONS 1. Dark matter indications Introduction: observing matter by gravity. Analogy with the discovery of Neptune. 1.1. Dispersion velocities of galaxies in clusters • First historical indication for dark matter (1933) • Virial theorem: relation between kinetic and potential energy • Derivation of Coma cluster mass following F. Zwicky paper [1]. The derived mass is 400 times larger than the observed mass 1.2. Rotation curves • Measurement of rotation velocity from the Doppler shift of the 21 cm hydrogen line • Expectation: decreasing velocities for increasing distance to the galactic centre q (outside the main visible mass distribution / 1=r) • Measurement: rotation curves are approximately flat for large radii • Early measurement in 1978 by V. Rubin [2], see figure1 (left) • A halo of additional 'dark' matter with density ρ / 1=r2 can explain the data at large radii • Recent detailed fitting of visible mass components and dark matter, see figure1 (right) and [3] Figure 1. Examples of rotation curve measurements. (Left) Figure from V. Rubin et al., 1978 [2]. (Right) Figure from E. E. Richards et al., arXiv:1503.05981 [3]). 1.3. Gravitational lensing • Deflection of light by a massive object (lens) as it travels from the source to the observer 4 1 DARK MATTER INDICATIONS • By analysing deflection patterns, the matter distribution of the lens can be reconstructed • Effect proposed by Einstein in 1936 [4] • Types of lensing [5]: strong, flexion, weak and microlensing. See figure2 for the classification of images from the first three lensing types Figure 2. Various regimes of gravitational lensing image distortion. A circular source is distorted into an ellipse by weak lensing being the typical resulting axis ratio ∼ 2 % (exaggerated for illustration). For most curved space-time (most massive objects), strong gravitational lensing produces multiple imaging and giant arcs. Figure from R. Massey et al. (2010), arXiv:1001.1739 [5]. • Strong lensing for dense concentrations of mass. If source is behind, Einstein rings can appear, otherwise multiple images result. See figure3 for observed examples Figure 3. (Left) Strong lensing: Einstein ring. Figure from ESA/Hubble & NASA. (Right) Weak lensing in Abell 2218. Figure from NASA. • Weak lensing appears when the lines of sight pass through more extended objects. By analysing a large number of object behind the lens, a statistical analysis can be performed to extract a mass distribution map. See figure4 • Flexion is an intermediate effect between strong and weak lensing • Microlensing: small increase in observed luminosity when a small object crosses the line of sight of a star. Used to search for Jupiter-like objects, see also section 2.1.2 5 1 DARK MATTER INDICATIONS Figure 4. Weak lensing. (Left) Physical gravitational lensing produce E-modes, tangential to mass over densities. Other spurious artefacts can mimic E-modes. The curl-like B-mode is used to verify the E-mode resulting potential. (Right) Observed ellipticity of half a million galaxies within 2 square degree Hubble COSMOS survey. Lines represent the mean ellipticity. From R. Massey et al. (2010), arXiv:1001.1739 [5]. 1.4. Galaxy-cluster collisions • Galaxy clusters are composed by stars in galaxies, gas clouds and dark matter • Rare events: collision of two such objects (tangential to observers on Earth) • X-ray measurements determine the collision of the gas clouds which are the main visible-mass constituent of the galaxy cluster • Telescope in the 'visible' range determine the position of galaxies • Weak gravitational lensing used to determine the total mass distribution • Few examples of such event are shown in figure5, see also reference [6] Figure 5. Galaxy-cluster collisions: bullet cluster, Abell 520 and DLSCL J0916.2+2951, respectively. Figures from astro-ph/0608407 [6], X-ray: NASA / CXC/ U. Victoria/ A. Mahdavi et al. and arXiv:1110.4391. • Recent study of 72 cluster collisions [7] used to determine the average displacement of dark matter center to the baryonic matter centre, see figure6 6 1 DARK MATTER INDICATIONS • Limits on the dark matter self-interaction can be placed [7] Figure 6. Galaxy-cluster collisions: (Right) Diagram of the displacement between gas (red), dark matter (blue) and the stars (green). (Left) Displacement results for 72 collisions. Figure from D. Harvey et al. Science 347 (2015) 1462, arXiv:1503.07675 [7]. 1.5. Cosmic microwave background • CMB: thermal photon radiation emitted at the end of recombination era • Isotropic emission with a temperature of T = 2:7 K • Predicted by Gamov ∼ 1950 • First measurement by Penzias and Wilson, accidentally, in 1964 using horn antennas for telecommunications • CMB anisotropies: fist measured by the COBE satellite in 1992. Also confirmed the black-body shape of the spectrum Figure 7. Cosmic microwave background maps. (left) Figure Planck Collaboration, arXiv:1507.02704 [8]. (Right) CMB resolution for different satellite measurements. • WMAP and Planck satellites measured the anisotropies (10−5 K) in great detail. See figure7 for a current map [8] (left) and the comparison of the resolutions (right) 7 1 DARK MATTER INDICATIONS • Most recent measurements by the Planck satellite operated from 2009 to 2013 • Two instruments (low and high frequency) to cover different frequency regions • The backgrounds of the measurement affect differently the various frequencies, see in figure8 (left) the different backgrounds to the measurement and the frequencies measured by Planck (grey vertical bands) Figure 8. (left) CMB foregrounds as modelled by the Planck experiment. Figure from Planck
Details
-
File Typepdf
-
Upload Time-
-
Content LanguagesEnglish
-
Upload UserAnonymous/Not logged-in
-
File Pages56 Page
-
File Size-