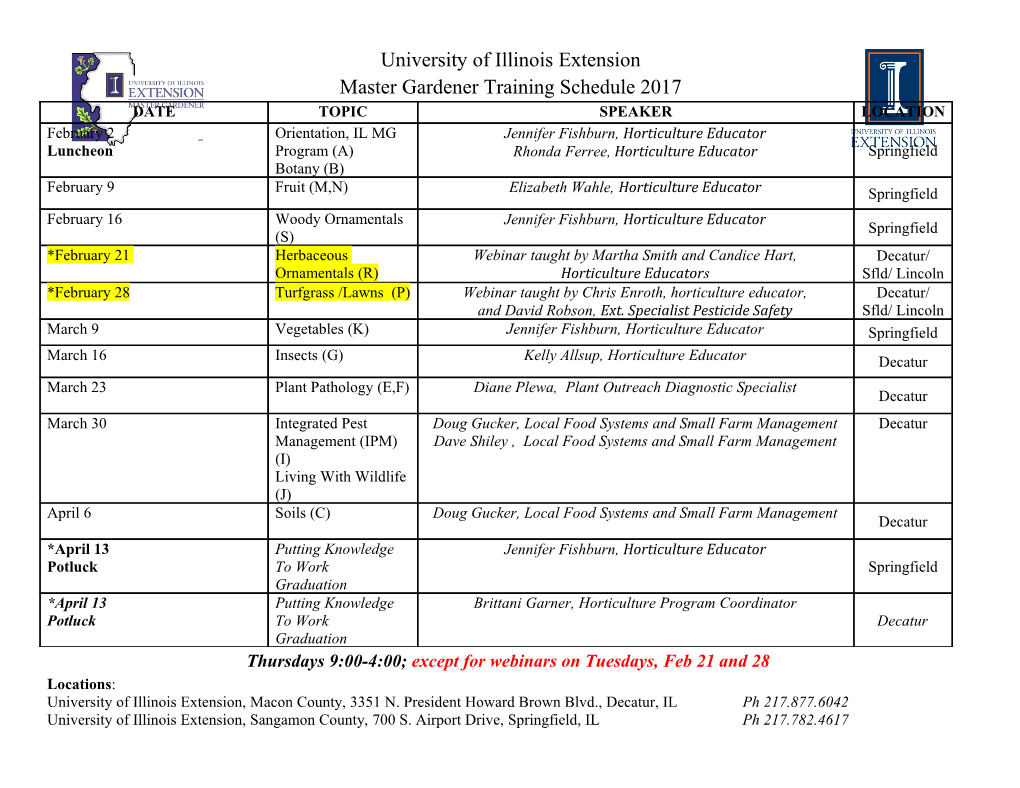
EXTRACTING PHYCOCYANIN FROM SPIRULINA AND HYDROTHERMAL LIQUEFACTION OF ITS RESIDUES TO PRODUCE BIO-CRUDE OIL BY SHAOCHEN GUAN THESIS Submitted in partial fulfillment of the requirements for the degree of Master of Science in Agricultural and Biological Engineering in the Graduate College of the University of Illinois at Urbana-Champaign, 2016 Urbana, Illinois Master’s Committee: Professor Yuanhui Zhang, Chair Professor Marcio Arêdes Martins Dr. Lance Charles Schideman ABSTRACT Microalgae is a promising biofuel source with high photosynthetic efficiency, and it often contains high-value substances for nutritional, pharmaceutical and medical applications. In order to improve the economic viability of the spirulina utilization, this study investigated a cost competitive biotechnological process for C-phycocyanin (C-PC) extraction from spirulina platensis. The residual biomass after C-PC extraction was converted into bio-crude oil via hydrothermal liquefaction (HTL). The HTL bio-crude oil obtained from the original spirulina feedstock and the residual after the C-PC extraction were compared. A rapid and efficient process for extraction and purification of food-level C-PC from spirulina platensis was developed in this study. The process conditions include: 1) freeze the spirulina 3 hours to extract the crude protein; 2) add 80 g/L (w/v) activated carbon to the crude extract; 3) use a vacuum filter with a 0.22 μm pore size membrane to collect the extract; and 4) freeze dry the extract to get the C-PC powder. The yield of the C-PC production is 27% and the cost (exclude labor) for entire process is $26.1/kg. After C-PC extraction, there is still 63% dry biomass left which was used for conversion into bio-crude oil via HTL. The HTL bio-oil products distribution as well as their composition, reaction pathways and energy recovery via HTL were investigated. HTL was conducted at temperatures range from 260˚C to 300˚C at 0.7 MPa N2 initial pressures. The highest bio-crude oil yield of 38 % (based on dry volatile matter) was obtained at 300˚C. The highest higher heating value is 37.1 MJ/kg occurred at 300°C reaction temperature. Elemental analysis revealed that the decarboxylation and denitrification may be dominant from 260˚C to 300°C following repolymerization governing at higher temperature; TG analysis showed that approximately 75.4 % distilled bio-crude products were in the range of 200-550°C. These distillates can be further upgraded for transportation fuels. ii To My Mother, Father, Grandparents iii ACKNOWLEDGMENTS First and foremost, I would like to appreciate sincerely to my advisor, Professor Yuanhui Zhang, for his patient discussion, always-positive encouragement, generous support and always kind help during the past three years. Without his help and guidance, I cannot complete this thesis. Working with him, I have improved and learned tremendously both in research and engineering skill. I feel fortunate to be part of his group. The appreciation also goes to Professor Marcio Arêdes Martins and Dr. Lance Charles Schideman. Thanks for their kind help on serving as my committee members and giving me valuable feedbacks. Without their help and advice, this work cannot be accomplished in a good manner. Also, I would like to deeply thank all my co-workers, especially Mr. Steven Ford and Dr. Yigun Sun. Thanks for their help on maintaining reactors as well as patient discussion. My sincere gratitude also goes to fellow graduate students: Grace Chen, Hao Li, Peng Zhang and Michael Stablein. I benefited significantly from their valuable discussion and shared experience. Only on their shoulders, I can see far about my research project. At last but not least, I would like to sincerely appreciate all my family, especially my grandfather, grandmother and my parents. I deeply appreciate my grandfather’s enlightening, teaching and taking-care of me as my life advisor. I want to express my deep gratitude to my Mother and Father. Thanks for your selfless support, love and cultivation. I love you and miss the days living closer to you. My appreciation also goes to my friends and colleagues here in UIUC. iv TABLE OF CONTENTS CHAPTER 1. INTRODUCTION………………………...…………..…….………………….......1 CHAPTER 2. LITERATURE REVIEW…………………......……….………………...…………4 CHAPTER 3. EXPERIMENTAL METHODS………………..…………………………………18 CHAPTER 4. EXTRACTION AND PURIFICATION OF PHYCOCYANIN FROM SPIRULINA……………………………………………………………………………………...29 CHAPTER 5. HTL OF RESIDUES AFTER PHYCOCYANIN EXTRACTION……….....…....46 CHAPTER 6. CONCLUSION………………………………...……….………….………..……71 REFERENCES ..............................................................................................................................74 APPENDIX A. A TYPICAL TEMPERATURE INCREASE/DECREASE PROFILE OF 100 ML BATCH REACTORS…………………………………………………………..……………...…80 v CHAPTER 1. INTRODUCTION 1.1 BACKGROUND With consumers growing interest in natural and healthy products, microalgae have been recognized as a source of functional ingredients with positive health effects due to these microorganisms produce polyunsaturated fatty acids, polysaccharides, natural pigments, essential minerals, vitamins, enzymes and bioactive peptides (Cuellar-Bermudez et al., 2015). These valuable compounds are very expensive, Table 1.1 shows some different products based on micro algal high-value molecules and their price in the global market (Spolaore et al., 2006a). Phycobiliproteins (PBPs) are a family of light-harvesting pigment proteins found in cyanobacteria and red algae, and there are two types of PBPs presented in the cyanobacterium spirulina (Arthrospira) platensis, the major type is C-phycocyanin (C-PC), while Allophycocyanin (APC) is less abundant. Together they count for up to 60% of the total cellular protein content (Yan et al., 2010). Because of C-PC excellent spectroscopic properties, stability, high absorption coefficient and high quantum yield, there is a wide range of promising applications of C-PC in biomedical research, diagnostics and therapeutics. In addition, it has potential as natural colorants for use in food, cosmetics and pharmaceuticals, particularly as substitutes for synthetic dyes, which are generally toxic or otherwise unsafe (Bermejo et al., 2003b). C-PC has wide usage and great economic potential. However, the widespread use of C- PC has been somewhat limited by the high cost of purification: For uses as biomarker or 1 treatment, the cost was ranging from $10 to 50 per mg; For uses as colorant in food industry this price reduces to $1–5 per gram because lower purity is requested (Ramos et al., 2011). Table 1.1 Prices of different products based on microalgal high-value molecules (Spolaore et al., 2006b) Product name Price (US$) Distributor R-phycoerythrin 3.25–14/mg Cyanotech Allophycocyanin 6–17/mg Cyanotech Streptavidin: B-phycoerythrin 145/mg Martek Goat anti-mouse IgG: R-phycoerythrin 165/mg Martek Sensilight PBXL1: anti GST 1,500/mg Martek Mixed fatty acids 60/g Spectra Stable Isotopes C-mixed free fatty acids 200/g Spectra Stable Isotopes C-DHA (>95%) 38,000/g Spectra Stable Isotopes N-alanine 260/g Spectra Stable Isotopes H7, C, N4-arginine 5,900/g Spectra Stable Isotopes dATP-CN 26,000/g Spectra Stable Isotopes Microalgae have unique advantages of being feedstocks for biofuels, such as high photosynthetic efficiency, non-arable land use, and tremendous environmental benefits through the capture of CO2 and nutrients (Biller & Ross, 2011; Duan & Savage, 2011b). Current algae- to-biodiesel technologies mainly focused on utilizing high-lipid algae and extracting lipids for biodiesel production (Yu et al., 2011). Wet biomass requires energy-intensive drying processes to remove excess moisture. High-lipid content algae species typically have much lower biomass productivity than low lipid content algae species. In comparison, hydrothermal liquefaction 2 (HTL) can directly convert low-lipid, fast-growing and wet algae biomass into a liquid bio-crude oil with or without a catalyst (Ross et al., 2010; Yu et al., 2011), carried out in a closed reactor at 200–350 °C and 5–15 MPa. HTL is regarded as one of the most promising methods for converting biomass into biofuels due to the non-requirement of drying feedstock and the efficient reaction medium (water) at high temperature and high pressure (Vardon et al., 2012). 1.2 OBJECTIVE Spirulina, a common fast-growing low-lipid green microalgae, has been researched as a feedstock for HTL experiment. However, the high cultivation costs of spirulina restrict the possibility of its mass production of crude oil. As the C-PC is a high value pigment in spirulina, and after its extraction there are amount of solids residual left, it is suggested to extract the C-PC from the spirulina firstly, then use the residues as the feedstock in HTL to achieve the goal of reducing the cost of feedstock in HTL. The objectives of this study are two folds: 1) Develop a cost competitive biotechnological process for extraction of C-PC from spirulina; and 2) Utilize the spirulina residues after C-PC extraction as a HTL feedstock to produce bio- crude oil. Effects of the reaction temperature and different feedstocks on the bio-crude oil yields as well as the composition of the liquefaction products are analyzed. Characterization including elemental analysis, GC-MS, and TGA for the liquefaction products is applied to examine the nutrients recovery, physiochemical properties and possible reaction pathways for bio-crude oil formation. This approach will substantially improve the economic viability of the
Details
-
File Typepdf
-
Upload Time-
-
Content LanguagesEnglish
-
Upload UserAnonymous/Not logged-in
-
File Pages85 Page
-
File Size-