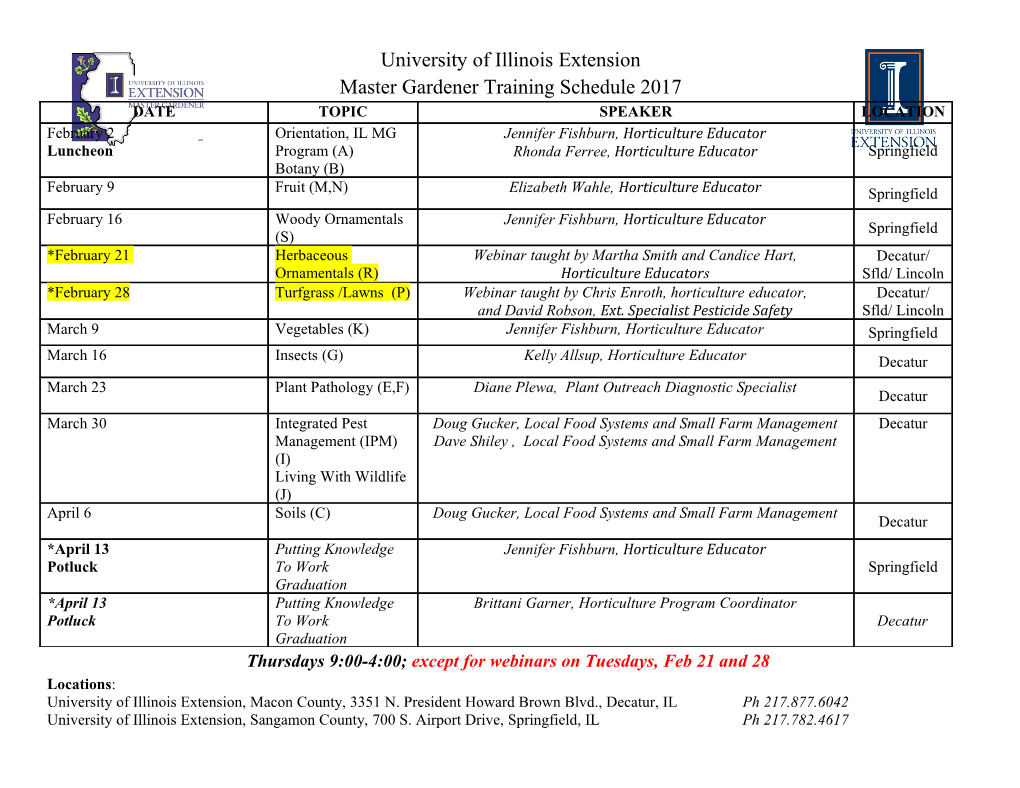
Vegetation Responses to Rapid Climate Change at the Late- Glacial/Holocene Transition John Birks Nordforsk PhD course, Abisko 2011 Why is a Quaternary-time palaeoecological perspective relevant to questions of migration, persistence, and adaptation? How did biota respond to past rapid climate change? Younger Dryas/Holocene transition at 11,700 calibrated years BP at Kåkenes Terrestrial vegetation and landscape development Possible modern analogues Chironomid-inferred temperatures and delayed arrival of Betula Other biotic responses at the YD/H transition Lake development and aquatic changes Conclusions 1 What could have determined persistence, migration, or extinction in the past? How can Quaternary palaeoecology provide insights to understanding migration and persistence? What conclusions can Quaternary palaeoecology draw about vegetation dynamics? Ecosystem functional change at YD/H transition Novel ecosystems Conclusions Why is a Quaternary Palaeoecological Perspective Relevant? Long argued that to conserve biological diversity, essential to build an understanding of ecological processes into conservation planning Understanding ecological and evolutionary processes is particularly important for identifying factors that might provide resilience in the face of rapid climate change Problem is that many ecological and evolutionary processes occur on timescales that exceed even long-term observational ecological data-sets ( ~100 yrs ) 2 Challenge for palaeoecological studies is to obtain the temporal resolution of documentary records and observational data. Needed if we are to evaluate biotic responses to rapid climate changes that may have occurred over 20-50 years and may occur in the future. (Modified from Oldfield 1983) Dawsonal. et (2011) Integrated approach to climate-change biodiversity assessment 3 Dawsonal. et (2011) Modes of biotic response to environmental change Very useful framework to view biotic responses Major step forward “Drawing on evidence from palaeoecological observations , recent phenological and microevolutionary responses, experiments, and computational models, we review the insights that different approaches bring to anticipating and managing the biodiversity consequences of climate change, including the extent of species’ natural resilience.” Dawson et al. (2011) 4 One approach for dealing with the data-gap between ecological and evolutionary time-scales is to rely on modelling . These models focus on future spatial distributions of species and assemblages under climate change rather than the ecological responses to climate change. Many crippling assumptions and serious problems of scale. Strongly dismissed by Dawson et al. (2011). High-resolution palaeoecological records provide unique information on species dynamics and their interactions with environmental change spanning 100s or 1000s years. How did Biota Respond to a Past Rapid Climate Change? Do biota migrate, persist, adapt, or go extinct locally or regionally? The end of the Younger Dryas at 11700 years ago is a perfect ‘natural experiment ’ for studying biotic responses to rapid climate change 5 North Greenland Ice Core Project (NGRIP) Subannual resolution of δ18 O and δD, Ca 2+ , Na +, and insoluble dust for 15.5-11.0 ka with every 2.5-5 cm resolution giving 1-3 samples per year. Used ‘ramp-regression’ to locate the most likely timing from one stable state to another in each proxy time-series. Steffensen et al . 2008 Science 321: 680-684 YD/Holocene at 11.7 ka deuterium excess (d) ‰ δ18 O ‰ log dust log Ca 2+ log Na + layer thickness ( λ) Annual resolution Ramps shown as bars Steffensen et al . 2008 6 δ18 O – proxy for past air temperature: YD/H 10ºC in 60 yrs annual layer thickness (λ): increase of 40% in 40 yrs d = δD – 8δ18 O (deuterium excess) – past ocean surface temperature at moisture source: changes in 1-3 yrs Dust and Ca 2+ - dust content: decrease by a factor of 5 or 7 within 40 yrs (plots are reversed) Na +: little change Indicate change in precipitation source ( δD) switched mode in 1- 3 yrs and initiated a more gradual change (over 40-50 yrs) of Greenland air temperature Changes of 2-4ºK in Greenland moisture source temperature from 1 year to next Ice-cores show how variable the last glacial period was – no simple Last Glacial Maximum Younger Dryas/Holocene Transition at 11,700 Calibrated Years BP 1. Remarkable climatic shift and rapid warming event felt over much of the Earth's surface 2. 'Global change' by any definition 3. Represents a global 'natural experiment' allowing us to investigate biotic responses to rapid climatic change ‘Coaxing history to conduct experiments’ Deevey (1969) ‘Using the geological record as an ecological laboratory’ Flessa & Jackson (2005) 7 Kråkenes Lake, Western Norway 8 Kråkenes Lake and cirque with YD moraine in Mehuken Mountain moraine Coring 9 Kråkenes cores at the YD/Holocene transition Detailed study of the Younger Dryas-Early Holocene transition designed to answer the following • What were the biological responses? • What happened on land and in the lake? • How does the Kråkenes vegetational development compare with vegetational changes today? • What were the rates of change and the magnitude of compositional turnover (beta-diversity)? • What factors may have controlled the terrestrial vegetational development? Part of multidisciplinary study of Kråkenes Lake led by Hilary Birks 10 Palaeoecological Data 1. Pollen analysis by Sylvia Peglar 600-769.5 cm 117 samples 101 taxa 16 aquatic taxa 2. Macrofossil analysis by Hilary Birks Pollen analyses supplemented by plant macrofossil analyses that provide unambiguous evidence of local presence of taxa, for example, birch trees 3. Diatom analysis Aquatic changes in the lake studied by fine resolution diatom analyses by Emily Bradshaw 4. Chironomid analysis Past temperatures estimated from fossil chironomid assemblages by Steve Brooks and John Birks 5. Radiocarbon dating by Steinar Gulliksen Chronology based on 72 AMS dates, wiggle-matched to the German oak-pine dendro-calibration curve by Gulliksen et al . (1998 The Holocene 8: 249-259) 6. Pollen sample resolution Mean age difference = 21 years Median age difference = 14 years Chronology in calibrated years is the key to being able to put the palaeoecological data into a reliable and realistic time scale 11 Kråkenes Early Holocene pollen sample resolution 12000 11500 11000 10500 10000 Age (calibrated years BP) 9500 9000 600 650 700 750 800 Depth (cm) Terrestrial vegetation and landscape development Krakenesº Major plant types only Early Holocene - Summary o C 0 5 5 at n io um nit S tes n hy n-ig p io o s o rb rid e te Lithology Loss- H P Dwarf-shrubs Trees & Shrubs Calculat Algae Aquatics Zone 9200 600 610 9400 620 630 9600 640 9800 650 7 10000 660 670 10200 10400 680 10600 690 10800 Depth(cm) 700 Calibrated years BP years Calibrated 710 6 11000 720 5 730 11200 740 4 11400 750 3 760 2 11600 1 770 20 40 20 40 60 80 20 40 20 20 40 60 100 200 300 400 500 20 40 60 80 100 20 Percentages of Calculation Sum 12 Zone 1 Younger Dryas – herb-dominated, no aquatics or Zone 1/2 Younger Dryas–Holocene transition at 11550 Zone 2 Earliest Holocene – spread of Zone 3 Major expansion of algae and beginnings of aq Zone 4 Beginnings of expansion of ferns Major changes macrophytes Zone 5 Expansion of dwarf shrubs and beginning of de YD Zone 6 Shrubs and some birch trees start to rise algae 50 years Zone 7 Tree, shrub, and fern dominance after end of YD370 years after end of YoungerSalix Dryas YD (willow) communities after end of YD yr BP algae Krakenes 110 years Early Holocene - Major Taxa ° 9200 after end of uatic 9400 600 9600 610 9800 620 Lithology 10000 630 Loss-on-ignition at 550 oC 640 10200 10400 650 720 years 10600 660 Saxifraga oppositifolia-type cline of 10800 670 Calibrated years BP Ranunculus glacialis-type 680 11000 Sedum 690 Depth (cm) 575 years 700 11200 Capsella-type 710 11400 720 Rumex acetosella-type 730 after end of 11600 740 750 Koenigia islandica 760 Oxyria digyna 770 Salix undiff. Two statistically significant pollen zone boundarie YD, 3 zone boundaries in 370 years, 4 zone boundari Salix herbacea-type 5 zone boundaries in 20720 40 years (first expansion of Gramineae Carex-type Very rapid Dryopteris-type dynamics. 20 Percentages of Calculation Sum 20 Filipendula 20 Rumex acetosa Empetrum nigrum pollen stratigraphical changes and hence Juniperus communis Betula 20 40 G mnoc p um dry ter s 20 y ar i op i Polypodium vulgare agg. 20 Populus tremula Pinus sylvestris 20 Corylus avellana or s .a ar 20 40 S bu cf. S ucup ia Zone 20 20 7 s in 110 years20 40 since es in 575 years, and Betula 6 5 20 4 rapid vegetational). 20 3 2 1 13 Kråkenes terrestrial macrofossils – summary diagram Analysed by Hilary H. Birks a ) e it p s angi u (fr a ty tosa i por pi ed ens eaf sc ea rmed eae s se l gyna e acea leave Cyr B P os b iac r di int ni gra t ype um 1 4 a trum tr fraga ces ia n od e e yr ix her am ineae yp ula pubeula fruit (tree) axi edum agi al ol m p m p et et Cal Saxifraga rivulS arisS Ox S S Gr P Carex E E B B Depth(cm) 665 670 675 Years 680 685 since 690 695 YD/Hol 700 10,870 705 720 10,920 710 670 715 720 575 725 11,180 730 370 735 11,270 740 290 11,385 745 110 750 EH 755 11,530 760 765 YD 770 20 40 20 20 20 500 1000 1500 20 40 1000 2000 5010 0 20 20 40 20 20 40 60 Analyst: Hilary Birks Krakenes° - Mineral Residue Landscape 100 changes – 95 90 became 85 increasingly 80 75 more stabilised 70 65 Mineral residue % within 300 years 60 after YD 9000 9500 10000 10500 11000 11500 12000 Age (calibrated years BP) Krakenes° - Loss-on-Ignition 40 35 30 25 20 15 10 5 Loss-on-Ignition % 0 9000 9500 10000 10500 11000 11500 12000 Age (calibrated years BP) 14 Terrestrial vegetation and landscape development Zone Age (cal Years yr BP) since YD 7 Betula woodland with Juniperus , Populus , Sorbus aucuparia , and later Corylus .
Details
-
File Typepdf
-
Upload Time-
-
Content LanguagesEnglish
-
Upload UserAnonymous/Not logged-in
-
File Pages54 Page
-
File Size-