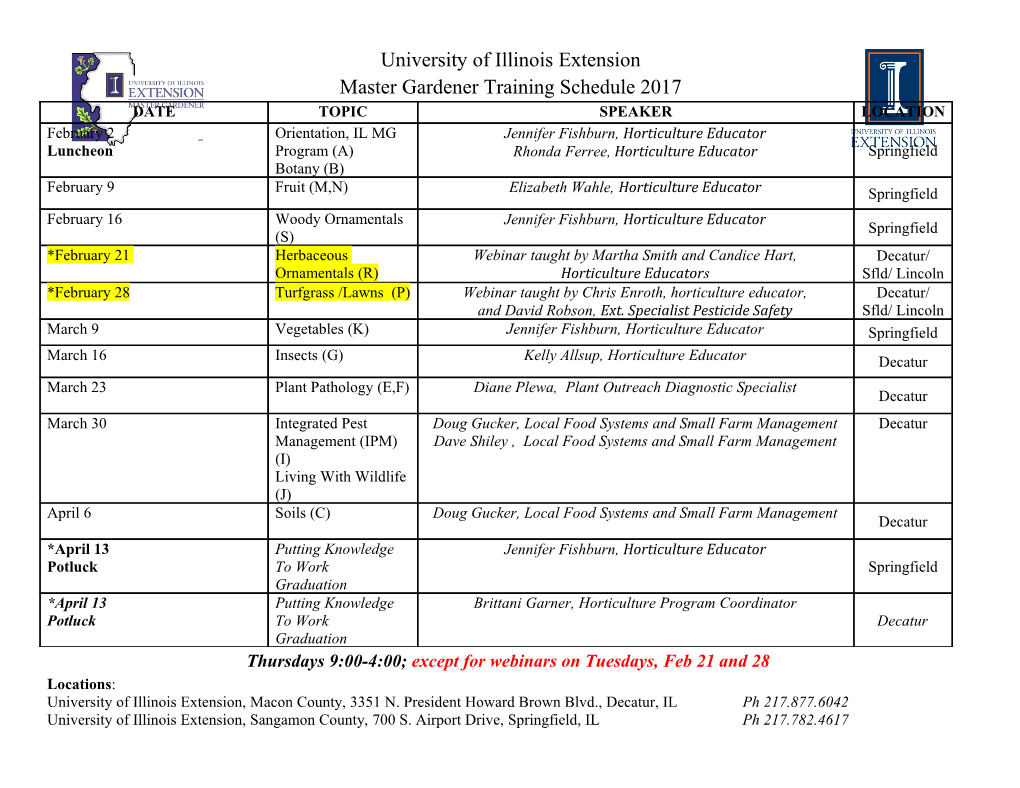
Journal of Industrial Microbiology & Biotechnology https://doi.org/10.1007/s10295-018-2021-9 METABOLIC ENGINEERING AND SYNTHETIC BIOLOGY - ORIGINAL PAPER Synthetic biology of polyketide synthases Satoshi Yuzawa1,2 · Tyler W. H. Backman1,2,3 · Jay D. Keasling1,2,3,4,5,6 · Leonard Katz2,3 Received: 8 December 2017 / Accepted: 3 February 2018 © Society for Industrial Microbiology and Biotechnology 2018 Abstract Complex reduced polyketides represent the largest class of natural products that have applications in medicine, agriculture, and animal health. This structurally diverse class of compounds shares a common methodology of biosynthesis employing modular enzyme systems called polyketide synthases (PKSs). The modules are composed of enzymatic domains that share sequence and functional similarity across all known PKSs. We have used the nomenclature of synthetic biology to classify the enzymatic domains and modules as parts and devices, respectively, and have generated detailed lists of both. In addition, we describe the chassis (hosts) that are used to assemble, express, and engineer the parts and devices to produce polyketides. We describe a recently developed software tool to design PKS system and provide an example of its use. Finally, we provide perspectives of what needs to be accomplished to fully realize the potential that synthetic biology approaches bring to this class of molecules. Keywords Biosynthesis · Cheminformatics · Natural products · Novel chemicals · Refactoring · Streptomyces Introduction including standardized, of the shelf parts (e.g., genes) with standardized connections that could be used and reused in Synthetic biology is an emerging discipline that combines the construction of devices and larger systems. For example, the felds of biology, biotechnology and engineering for the a computer can be assembled from standardized parts and design and construction of new biological parts, devices, and devices (sound cards, processors, operating systems, hard systems, as well as the re-design of existing natural biologi- drives, etc.) available from many vendors; devices such as cal systems, all for useful purposes. To make the engineering monitors, scanners, printers, etc., are connected to the com- of biology faster, more reliable, and more reproducible, syn- puter through a standard port. thetic biologists have developed a framework for engineer- Synthetic biology has numerous applications: it can be ing biology that is similar to other engineering disciplines, used for the construction of proteins that can serve as new vaccines, or new biochemical pathways synthesizing small molecules that can serve as pharmaceuticals, chemicals (sol- Satoshi Yuzawa and Tyler W. H. Backman contributed equally. vents, adhesives, food favorings, cosmetics, etc.), or bio- fuels, plant and animal growth stimulators or insecticides. * Leonard Katz [email protected] Additional applications include the generation of new bio- sensors for early detection of medical conditions, or to detect 1 Biological Systems and Engineering Division, Lawrence environmental pollution. The hosts used for production of Berkeley National Laboratory, Berkeley, CA 94720, USA new proteins, small molecules, or biosensors were desig- 2 Joint BioEnergy Institute, Emeryville, CA 94608, USA nated as chassis. Synthetic biology also includes the direct 3 QB3 Institute, University of California, Berkeley, CA 94720, engineering of chassis, as well as the technologies such as USA CRISPR/Cas9 to make chassis easier to engineer. 4 Department of Bioengineering, University of California, Reduced polyketides are a sub-class of natural products Berkeley, CA 94720, USA that have a variety of medical, veterinary, and agricultural 5 Department of Chemical and Biomolecular Engineering, applications. Examples, shown in Fig. 1, include the US University of California, Berkeley, CA 94720, USA Food and Drug Administration (FDA)-approved antibacte- 6 Novo Nordisk Foundation Center for Biosustainability, rial agents erythromycin, fdaxomicin, rifamycin and tylosin; Technical University of Denmark, Hørsholm, Denmark Vol.:(0123456789)1 3 Journal of Industrial Microbiology & Biotechnology O OH O N O O OH HO H O O O O O O N O O OH O NH2 O H O O HO O O O OH O R O O HO O O OH O H O O OH O O O H O O O O OH O HO OH O O OH Discodermolide OH H H N H H O O O N O O O O O OH OH HO H Tylosin N O Spinosad Rifamycin O OH O O O HO O OH H OH O O OH Disorazole C O 1 O O OH O HO O O H R OH O O O O O O O O HO HO OH Avermectin R O O Chalcomycin O H B1a CH O OH O N 3 B1b H HO O O O H Monensin R O A C2H5 O B CH3 R O N O OH Salinosporamide HO H OH OH OH O O O R = Cl, F, Br OH OH O OH OH OH OH OH HO N O H OH Rapamycin N H O O O O OH O OH O O O OOO Borrelidin O HO O S O H O H N OH N H N OH O N O O 2 H O HH OH OH O Erythromycin Amphotericin O Curacin A O OH HO O O Splenocin OH HO O O O O Cl HO O OH OH O O OH O O OH O O O O O O O O OH Cl O CO2H O R N O OH H H OH HO O O O O O OH O CO2H HO2C O HO Fidaxomicin (tiacumicin B) H OH R O O Tacrolimus (FK506) N Lovastatin OH Reveromycins O R ,, -Lipomycin H R 21-Methyl- lipomycin CH3 O O O R = S O , O N OH OH N O O N O HO HO P OH H OH O O OH O OH O OH O O O NH2 O O O O Epothilone R O O OH Methymycin A H Fostriecin B CH3 Geldanamycin Fig. 1 Structures of natural polyketides. The parts of the compounds determined by the corresponding polyketide synthase are highlighted in dark the antifungal agent amphotericin; the immunosuppressive Some of the other compounds shown in Fig. 1 (e.g., chal- agents rapamycin and tacrolimus; the cholesterol lowering comycin, fdaxomicin, methymycin, and tylosin) undergo agent lovastatin; the anthelmintic agent avermectin; the similar post-polyketide processing, whereas others, such insecticide spinsosad; and monensin, a growth promotant as splenocin and geldanamycin undergo acylation or car- for ruminants and coccidiostat in poultry. Curacin, disco- bamoylation, respectively. Discodermolide and epothilone dermolide, epothilone, geldanamycin, and salinosporamide undergo little or no further elaboration. Some polyketides have anti-cancer properties and are in clinical trials. With are connected to an amino acid or amino acid derivative the exception of lovastatin, which is produced by the fungus (e.g., epothilone, rapamycin, tacrolimus, and lipomycin) Aspergillus terreus, as well as a number of higher plants, all via a linked non-ribosomal peptide synthetase (NRPS). The of the compounds shown in Fig. 1 are produced by bacteria, polyketide backbone of monensin reforms into a polyether, in particular members of the mycelia-forming family called whereas part of the backbone of the reveromycin assumes a actinomycetes that includes the most common genus Strep- spiroketal confguration. tomyces, as well as the genera Dactylosporangium, and Sac- Although great structural diversity is observed for pol- charopolyspora, among others. Many members of the myxo- yketides, they share, as outlined below, an overarching simi- bacteria family also produce polyketides (e.g., epothilone, larity in their biosynthesis. This has drawn the attention of disorazole). Curacin is made in the cyanobacterium Lyngbya a variety of scientists interested in understanding the fun- majuscula. Discodermolide was isolated from a sponge, but damental basis of how these molecules are made and the it is believed that it is produced by a symbiotic bacterium. common and diferentiating features of their biosynthesis. In Although these compounds do not resemble each other, and addition, their medical and industrial importance has drawn have diferent biological targets, they are all made by a class the interest of pharmaceutical and industrial companies who of enzymes known as modular polyketide synthases (PKSs), desire to apply genetic approaches to change the structures which produce the polyketide backbones. An example of a of polyketides to improve their properties or generate chemi- modular PKS is 6-deoxyerythronolide B synthase (DEBS), cal diversity. which produces 6-deoxyerythronolide B (6-dEB), the pol- This paper is intended for people interested in synthetic yketide backbone of erythromycin (Fig. 2), which undergoes biology who may not be familiar with polyketides and post-polyketide processing (glycosidation, hydroxylation, PKSs. It is, therefore, not intended to be comprehensive O-methylation) to generate the fnal product erythromycin. with respect to the detailed enzymology of PKS or present 1 3 Journal of Industrial Microbiology & Biotechnology Fig. 2 Organization of the 6-deoxyerythronolide B synthase (DEBS) and 13 in the acyl chain attached to module 6 undergo a lactonization and structure of its product, 6-deoxyerythronolide B (6dEB). Step- reaction conducted by the TE domain to result in the production of wise growth of the polyketide chain of the polyketide chain is shown the macrolactone 6-dEB, which undergoes further biochemical pro- attached to the ACP domain of each module after full elaboration, cessing (not shown) to generate erythromycin (Fig. 1). Abbreviations: including stereochemistry, by modular functions. Modules are labeled ACP, acyl carrier protein; AT, acyltransferase; DH, dehydratase; ER, Load through Module 6 and are delineated by solid black lines. The enoyltransferase; KR, β-ketoreductase; KS, β-acyl ACP synthase; TE, proteins comprising DEBS are shown by arrows. The atoms labeled 1 thioesterase a complete review of the genetic engineering of PKS genes acyl-CoAs or carboxyacyl-CoAs as substrates. The num- that has been reported over the past 27 years. For that, the ber of modules in a given PKS determines the size of the reader is directed to a number of recent reviews [3, 4, 21, 38, resultant polyketide produced, and the specifc carboxy- 44].
Details
-
File Typepdf
-
Upload Time-
-
Content LanguagesEnglish
-
Upload UserAnonymous/Not logged-in
-
File Pages13 Page
-
File Size-