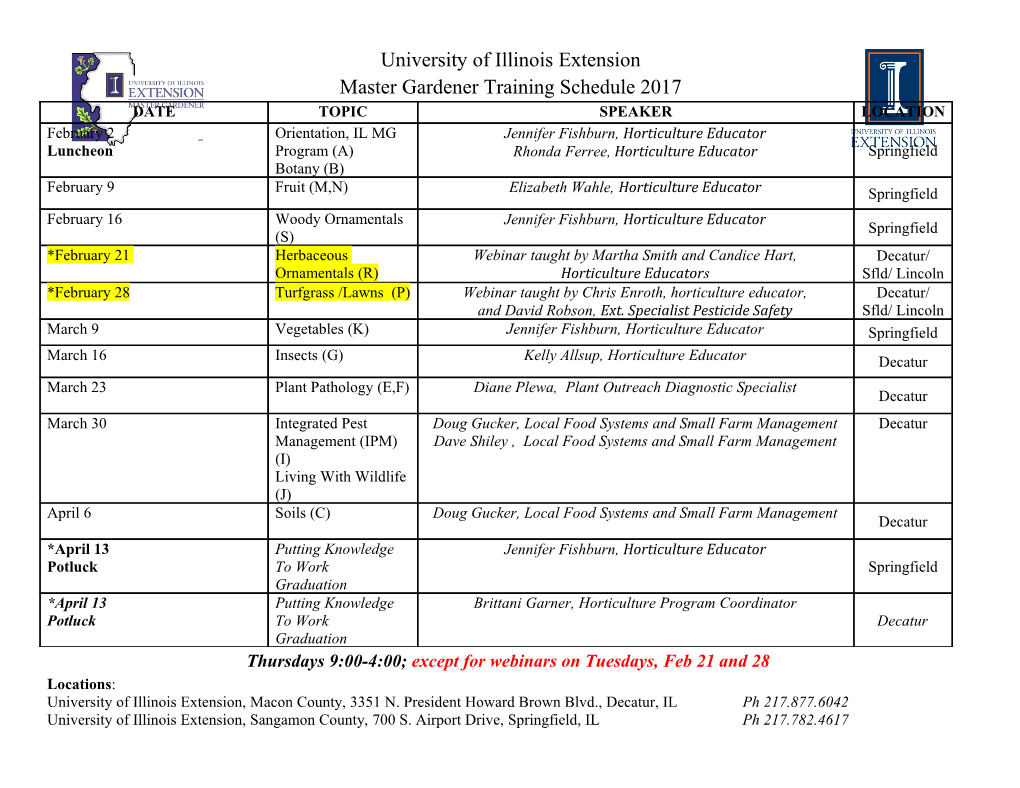
Environment International 129 (2019) 194–207 Contents lists available at ScienceDirect Environment International journal homepage: www.elsevier.com/locate/envint Review article Understanding the bioavailability of pyrethroids in the aquatic environment using chemical approaches T ⁎ Zhijiang Lua,b, , Jay Ganb, Xinyi Cuic, Laura Delgado-Morenod, Kunde Line a College of Environmental and Resource Sciences, Zhejiang Provincial Key Laboratory of Agricultural Resources and Environment, Zhejiang University, Hangzhou 310058, China b Department of Environmental Sciences, University of California, Riverside, CA 92521, United States c State Key Laboratory of Pollution Control and Resource Reuse, School of the Environment, Nanjing University, Nanjing 210046, China d Estación Experimental del Zaidín, Spanish National Research Council (CSIC), Granada, Spain e The Fujian Provincial Key Laboratory for Coastal Ecology and Environmental Studies, College of the Environment and Ecology, Xiamen University, Xiamen 361102, China ARTICLE INFO ABSTRACT Handling Editor: Robert Letcher Pyrethroids are a class of commonly used insecticides and are ubiquitous in the aquatic environment in various Keywords: regions. Aquatic toxicity of pyrethroids was often overestimated when using conventional bulk chemical con- Pyrethroid centrations because of their strong hydrophobicity. Over the last two decades, bioavailability has been re- Bioavailability cognized and applied to refine the assessment of ecotoxicological effects of pyrethroids. This review focuses on Tenax extraction recent advances in the bioavailability of pyrethroids, specifically in the aquatic environment. We summarize the Passive sampling development of passive sampling and Tenax extraction methods for assessing the bioavailability of pyrethroids. Risk assessment Factors affecting the bioavailability of pyrethroids, including physicochemical properties of pyrethroids, and quality and quantity of organic matter, were overviewed. Various applications of bioavailability on the as- sessment of bioaccumulation and acute toxicity of pyrethroids were also discussed. The final section of this review highlights future directions of research, including development of standardized protocols for measure- ment of bioavailability, establishment of bioavailability-based toxicity benchmarks and water/sediment quality criteria, and incorporation of bioavailability into future risk assessment and management actions. 1. Introduction example, Li et al. (2017) reviewed 58 studies on the occurrence of pyrethroids in sediment, and found that the detection frequencies for Pyrethroids are a class of commonly used insecticides. Their struc- bifenthrin, cyfluthrin, lambda-cyhalothrin, cypermethrin, esfenvale- tures are analogous to naturally-occurring pyrethrins found in the rate, and permethrin were 78, 37, 49, 48, 43, and 57%, respectively, flowers of Chrysanthemum, but with increased insecticidal potency as with the maximum concentrations reaching the μg/g range (dry weight, well as environmental stability. Because of their high efficacy and low dw). mammalian toxicity, synthetic pyrethroids have gained popularity for Although pyrethroids are known to have low mammalian toxicity, both agricultural and urban insect management in recent decades as they exhibit high levels of toxicity toward aquatic invertebrates and fish some organophosphate and carbamate insecticides were gradually (Nowell et al., 2016; Weston et al., 2015). For example, the median phased out (Kuivila et al., 2012; Lao et al., 2010). Although pyrethroids effective concentration (EC50) of bifenthrin for mayfly(Hexagenia sp.) are considered to be less recalcitrant to degradation than organo- immobilization was only 15.3 ng/L (Weston et al., 2015). The threshold chlorine insecticides, they can last for months in soils because of their effect benchmark of bifenthrin in freshwater sediment for midge enhanced chemical stability compared to pyrethrins, such as stability (Chironomus spp.) was only 0.55 μg/g-OC (Nowell et al., 2016). As a against photolysis (Meyer et al., 2013). As a result of their widespread result, pyrethroids have been acknowledged as a major contributor to use and relatively long persistence, residues of pyrethroids have been sediment (Cheng et al., 2017; Holmes et al., 2008; Weston et al., 2013; found ubiquitously in the aquatic environment in many regions in the Weston et al., 2005; Yi et al., 2015) and surface water toxicity (Wolfram world (Li et al., 2017; Moschet et al., 2017; Moschet et al., 2014; et al., 2018). Schreiner et al., 2016; Van Metre et al., 2017; Wei et al., 2017). For Traditionally, the bulk or total chemical concentrations have been ⁎ Corresponding author at: College of Environmental and Resource Sciences, Zhejiang University, Hangzhou 310058, China. E-mail addresses: [email protected], [email protected] (Z. Lu). https://doi.org/10.1016/j.envint.2019.05.035 Received 29 December 2018; Received in revised form 27 April 2019; Accepted 13 May 2019 Available online 23 May 2019 0160-4120/ © 2019 The Authors. Published by Elsevier Ltd. This is an open access article under the CC BY-NC-ND license (http://creativecommons.org/licenses/BY-NC-ND/4.0/). Z. Lu, et al. Table 1 Summary of the literature using passive sampling methods to obtain chemical activities of pyrethroids in the aquatic environment. Compound Passive sampler Matrix Duration Technique Ffree (%) Reference 8 Pyrethroids SR 500 μm × 50 mm × 100 mm Freshwater sediment 7 d (in situ) Equilibrium sampling with PRC; in Xu et al., 2018 LDPE 25 μm × 50 mm × 150 mm 30 d (ex situ) situ and ex situ 8 Pyrethroids PU 381 μm × 5 mm × 50 mm River water 4 d Equilibrium sampling with PRC; in 0.27–47 Liao et al., 2017 situ 8 Pyrethroids LDPE 25 μm × 20 mm × 200 mm River water 7 d Equilibrium sampling with PRC; in 3–83 Xue et al., 2017 situ Cypermethrin, permethrin POCIS with 0.2 g Oasis HLB sorbent River water 28 d Kinetic sampling Zhang et al., 2016 9 Pyrethroids SPMD, size unknown River water 2 months Equilibrium sampling with PRC; in (Hapke et al., 2016 situ 10 Pyrethroids SR 0.5 mm × 10 cm × 30 cm River water 14 d Kinetic sampling; in situ Moschet et al., 2014 Bifenthrin, permethrin LDPE 60 μm × 2.5 cm × 98 cm Water 10 d Kinetic sampling O'Brien et al., 2012 SR 410 μm × 2.5 cm × 92 cm Fenvalerate, allethrin, SPMD 2.9 cm × 50 cm DI water 20 d Kinetic sampling Sabaliunas et al., 1998 Bifenthrin PDMS-SPME 7 μm × 200 mm DI water with 5 mg/L 40 min Kinetic sampling; online 45–65 Delgado-Moreno et al., commercial DOC 2015 Permethrin LDPE, 2.6 cm × 1 m River water 14 d Equilibrium sampling with PRC; in Anderson et al., 2014 situ Cypermethrin GF/F filter coated with ethylene vinyl acetate 7 μm × 70 mm Sea water Up to 16 d Equilibrium sampling; in situ Tucca et al., 2014 195 diameter 8 Pyrethroids PDMS-SPME 100 μm, length unknown Estuary sediment 29 d Equilibrium sampling; in situ Greenstein et al., 2014 Bifenthrin, cis- permethrin PDMS-SPME 35 μm × 10 mm Freshwater sediment 1, 2, 3, and 20 d Equilibrium sampling with and Cui et al., 2013a without PRC; ex situ 6 Pyrethroids PDMS-SPME 10 μm × 200 mm River sediment 28 d Equilibrium sampling; ex situ 1.6–5.3 Li et al., 2013 9 Pyrethroids PDMS-SPME 10 μm × 150 mm Freshwater sediment 42 d Equilibrium sampling; in situ and ex Harwood et al., 2013b situ Bifenthrin PDMS-SPME 10 μm × 400 mm Soil and freshwater sediment 28 d Equilibrium sampling; ex situ Harwood et al., 2013a Permethrin PDMS-SPME 10 μm × 10 mm Freshwater sediment 28 d Equilibrium sampling; ex situ Ding et al., 2013 5 Pyrethroids SPME with etched stainless steel River water 30 min Kinetic sampling; online Jia et al., 2010 Permethrin PDMS-SPME 10 μm × 100 mm Freshwater sediment 3, 7, 14, 28 d Equilibrium sampling; ex situ Pehkonen et al., 2010 7 Pyrethroids PDMS-SPME 30 μm × 10 or 20 mm Freshwater sediments 20 d Equilibrium sampling; ex situ 2.3–20.7 Hunter et al., 2009 Permethrin PDMS-SPME 30 μm × 10 mm Freshwater sediments 20 min Kinetic sampling; online 0.4–13.0 Hunter et al., 2008 Permethrin PDMS-SPME 10 μm × 10 mm Freshwater sediments 28 d Equilibrium sampling; ex situ 0.3, 0.4, 2.1, 5.3 You et al., 2007 Permethrin, cyfluthrin PDMS-SPME 35 μm × 30 mm Surface waters 24 h Kinetic sampling; 43–98 Yang et al., 2007 fl μ – Bifenthrin, cy uthrin, PDMS-SPME 30 m × 20 mm Sediment porewater 24 h Kinetic sampling; 2.9 27 Xu et al., 2007 Environment International129(2019)194–207 fenpropathrin 9 Pyrethroids PDMS-SPME 30 μm × 20 mm Freshwater and marine 20 min Kinetic sampling; online 4.1 to 37; Bondarenko et al., 2007 sediment porewater 3.2 to 13.3 4 Pyrethroids PDMS-SPME 30 μm × 20 mm Freshwater and marine 15 min Kinetic sampling; online 1.7–4.4; Bondarenko et al., 2006 sediment porewater 1.1–2.9 Bifenthrin, cis- and trans- PDMS-SPME 30 μm × 20 mm Stream water and runoff 5–80 min Kinetic sampling; online 0.4–1.0; Liu et al., 2004 permethrin 10–27 Bifenthrin, cis-and trans- PDMS-SPME 30 μm × 20 mm River sediments 10 min Kinetic sampling; online Lee et al., 2003 permethrin Z. Lu, et al. Environment International 129 (2019) 194–207 used to develop toxicity benchmarks for aquatic contaminants. For se- The principles and differences of these two approaches were well de- diment, to account for the dominant role of organic carbon (OC) in scribed in Reichenberg and Mayer (2006) and Cui et al. (2013b) and are regulating phase distribution of hydrophobic contaminants, OC nor- out of the scope of this review. Both of these approaches have been used malized concentrations have also been used in establishing toxicity to characterize the bioavailability of pyrethroids in aquatic systems. thresholds and sediment quality criteria. However, pyrethroids are ex- tremely hydrophobic, with log KOW values ranging from 4.5 to 7.0 3.1. Passive sampling for measuring the chemical activity of pyrethroids (Laskowski, 2002), and log KOC values ranging from 5.08 to 6.04 (Gan et al., 2008).
Details
-
File Typepdf
-
Upload Time-
-
Content LanguagesEnglish
-
Upload UserAnonymous/Not logged-in
-
File Pages14 Page
-
File Size-