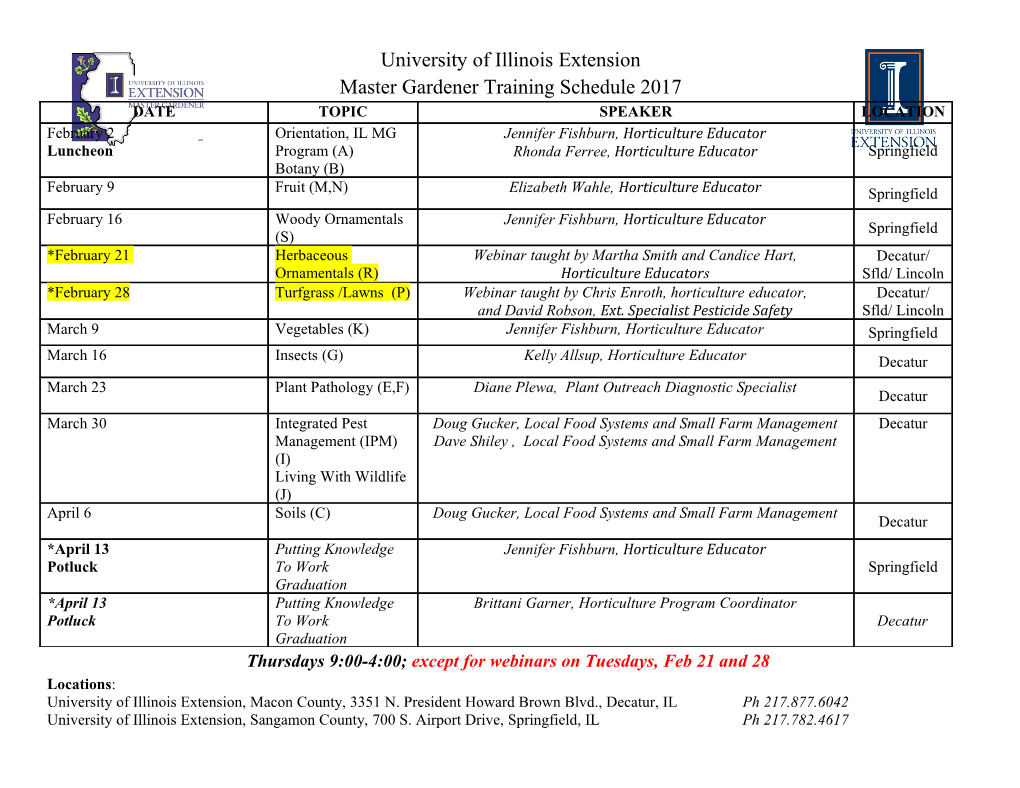
Downloaded from http://sp.lyellcollection.org/ by guest on September 27, 2021 Geology of the earthquake source: an introduction A˚ KE FAGERENG1* & VIRGINIA G. TOY2 1Department of Geological Sciences, University of Cape Town, Private Bag X3, Rondebosch 7701, South Africa 2Department of Geology, University of Otago, PO Box 56, Dunedin 9054, New Zealand *Corresponding author (e-mail: [email protected]) Abstract: Earthquakes arise from frictional ‘stick–slip’ instabilities as elastic strain is released by shear failure, almost always on a pre-existing fault. How the faulted rock responds to applied shear stress depends on its composition, environmental conditions (such as temperature and pressure), fluid presence and strain rate. These geological and physical variables determine the shear strength and frictional stability of a fault, and the dominant mineral deformation mechanism. To differing degrees, these effects ultimately control the partitioning between seismic and aseismic defor- mation, and are recorded by fault-rock textures. The scale-invariance of earthquake slip allows for extrapolation of geological and geophysical observations of earthquake-related deformation. Here we emphasize that the seismological character of a fault is highly dependent on fault geology, and that the high frequency of earthquakes observed by geophysical monitoring demands consider- ation of seismic slip as a major mechanism of finite fault displacement in the geological record. Rick Sibson has, throughout his career, pointed out the geological and physical parameters likely to that earthquakes occur in rocks (e.g. Sibson 1975, control their prevalence discussed. The mechanisms 1977, 1984, 1986, 1989, 2002, 2003). This simple by which rocks were deformed can be inferred from fact implies that fault rocks exert a critical control their textures (Knipe 1989); these relationships for on earthquake nucleation and propagation, and the typical fault rocks encountered in exhumed should contain an integrated record of earthquakes. fault zones are reviewed in this introduction. A con- Reading this record, Sibson has added significantly ceptual fault-zone model building on previous to our understanding of how faults work. His intui- review works (e.g. Sibson 1983; Scholz 2002) that tive ability to relate structures in exhumed rocks to is based on observations of the depth-distribution seismological observations of active deformation of fault rocks, inferred variability in deformation has contributed significantly to bridging the gap mechanism, fault strength and frictional stability between how geologists and geophysicists think with depth is also described. It is explained how about fault mechanics, and has led to the develop- this model provides basic controls on the depth ment of earthquake science as a truly interdisciplin- limits of the crustal seismogenic zone. Earthquake ary field. This volume celebrates Rick Sibson’s size and scaling parameters, in relation to interpret- career by bringing together a collection of papers ing data from different scales and methods, are also that present innovative studies spanning a range briefly reviewed; and, finally, the chapters in this of topics within the field of earthquake geology. Special Publication are outlined. Included are descriptions of fault-zone rocks from active and ancient faults, rock deformation exper- iments at seismic slip rates, and theoretical treat- Earthquake mechanism and frictional ments of fault initiation and slip. stability In this introduction the mechanism of earthquake faulting – generally accepted to be the release of Faults slip at a range of speeds, including slow creep tectonic elastic strain energy stored in a rock at plate tectonic rates (order of 1–10 cm year21), volume – by fast slip on a pre-existing or new fault fast frictional sliding (c.1ms21) during earthquake (Brace & Byerlee 1966) is discussed. Also included slip and displacement at a range of recently discov- is a short discussion on how rate- and state-variable ered, intermediate slip rates (such as slow slip and frictional stability may affect the rate at which fault low-frequency earthquakes: see the review by slip occurs (Dieterich 1972, 1979). The deformation Peng & Gomberg 2010 and references therein). mechanisms likely to be active at different con- The mechanisms of fault creep and slip at intermedi- ditions within a fault zone, which may lead to ate velocities are not yet obvious, but earthquake seismic or aseismic deformation, are outlined, and slip is generally thought to arise from ‘stick–slip’ From:Fagereng,A˚ ., Toy,V.G.&Rowland, J. V. (eds) Geology of the Earthquake Source: A Volume in Honour of Rick Sibson. Geological Society, London, Special Publications, 359, 1–16. DOI: 10.1144/SP359.1 0305-8719/11/$15.00 # The Geological Society of London 2011. Downloaded from http://sp.lyellcollection.org/ by guest on September 27, 2021 2 A˚ . FAGERENG & V. G. TOY frictional instability on a pre-existing fault (Brace & (Dieterich 1972, 1979; Scholz et al. 1972; Ruina Byerlee 1966) or, less commonly, by failure on a 1983; Marone 1998; Scholz 1998). Rate- and state- new fault surface. In this model, based on the variable effects on fault friction include an increase elastic rebound theory first suggested by Reid in ms with loading time, t, related to an increase (1911), elastic strain accumulates in rock surround- in real contact area with time under an applied ing the existing or potential fault surface (Fig. 1a), normal stress (Dieterich 1972). In addition, the until the resultant shear stress on the plane exceeds dynamic friction (mk), the sliding resistance experi- its frictional strength and failure occurs. Thus, earth- enced on a fault after slip has initiated, appears to quake faulting is a frictional process, where rupture vary with slip velocity, v (Fig. 1b) (Scholz et al. initiation depends on frictional resistance on the 1972). Although there are several forms of a consti- existing or potential fault plane. The shear stress tutive equation fitting laboratory observations of at failure, tf, is commonly approximated by the rock friction, a commonly used description of the empirical Amontons’ law: time and velocity dependence of friction is the Dieterich–Ruina law (Beeler et al. 1994): = + − tf C0 ms(sn Pf ) (1) = + + m m0 a ln(v/v0) b ln(v0u/D); where C is cohesive strength, m is the static coeffi- (2) 0 s du/dt = 1 − uv/D cient of friction, sn is normal stress and Pf is fluid pressure. Equation (1) indicates the shear stress con- ditions at which a fault will slip; however, it does not where m0 is the coefficient of friction at a reference define at what rate this slip occurs. slip velocity (v0), D is the critical slip distance, inter- Experimental studies of fault friction, generally preted as the slip increment needed for renewal of in homogeneous rock samples, have indicated that surface contacts (Dieterich 1979), a and b are empiri- a rate- and state-variable friction law can describe cal material constants, and u is a state variable observed sliding behaviour on laboratory faults that evolves over time. Geological effects, such as time Elastic strain (a) Start of new cycle Interseismic strain Post-seismic accommodation (b) Slow Fast 0.07 0.06 T2 b2 0.05 0.04 ° T1 = quartz at 150 C, velocity weakening Δm 0.03 ° T2 = quartz at 600 C, velocity strengthening a2 0.02 0.01 a1 T b1 0.0 1 -0.01 0 2 4 6 8 10 Slip distance/D Fig. 1. (a) Fault movement by elastic rebound. Starting from a relaxed state at the beginning of a seismic cycle, the rock surrounding the fault accumulates elastic strain. When this strain reaches a value where the resultant shear stress on the fault exceeds its frictional strength, displacement occurs on the fault, releasing stored elastic strain in the wall rock. (b) Example of the velocity dependence of friction, using material properties (a and b) for quartz from Blanpied et al. (1995) and Equation (2), at temperatures of 150 8C (solid line) and 600 8C (dashed line). Note the different evolution of the frictional coefficient (m) predicted for velocity-weakening and velocity-strengthening conditions. Downloaded from http://sp.lyellcollection.org/ by guest on September 27, 2021 GEOLOGY OF THE EARTHQUAKE SOURCE 3 mineralogy, fluid pressure, dominant deformation by fluid presence between grains (Carter 1975). mechanism and rock structure, as well as environ- Because of high porosity and, commonly, high mental variables including temperature and pressure, fluid content, diagenetic chemical processes such are here included in the empirical material constants as cementation in pore spaces, recrystallization and a, b and D, and in the time-dependent state variable, u. fluid-assisted diffusional mass transfer into sites of Stick–slip instabilities, proposed by Brace & low stress are also likely to contribute to this sort Byerlee (1966) to result in earthquake slip, may of deformation (Maltman 1994). These chemical only occur where the fault material exhibits unstable processes are unlikely to accommodate much dis- or conditional frictional stability (Scholz 2002). placement but, rather, lead to an increase in cohe- Frictional stability depends on the empirical con- sion and rigidity, suppressing granular flow and stants a, b and D; where, if the difference (a 2 b) increasing the ability of the rock to store elastic is positive, the material is said to be ‘velocity strain. A shallow transition from continuous granu- strengthening’ and m increases with slip (Fig. 1b). lar flow to frictional, discontinuous deformation of Such material is inherently stable and cannot slip cohesive rock capable of elastic rebound may there- at seismic velocities (Dieterich 1979). However, if fore occur in response to increasing confining (a 2 b) is negative, friction decreases with slip pressure, and to mechanical and chemical cementa- (Fig. 1b) and the material is ‘velocity weakening’. tion (Moore & Saffer 2001). In this case, the material is either unstable or con- ditionally stable; earthquakes may only nucleate in Grain-size-sensitive creep the unstable regime but can propagate into con- ditionally unstable regions (Scholz 1998).
Details
-
File Typepdf
-
Upload Time-
-
Content LanguagesEnglish
-
Upload UserAnonymous/Not logged-in
-
File Pages16 Page
-
File Size-