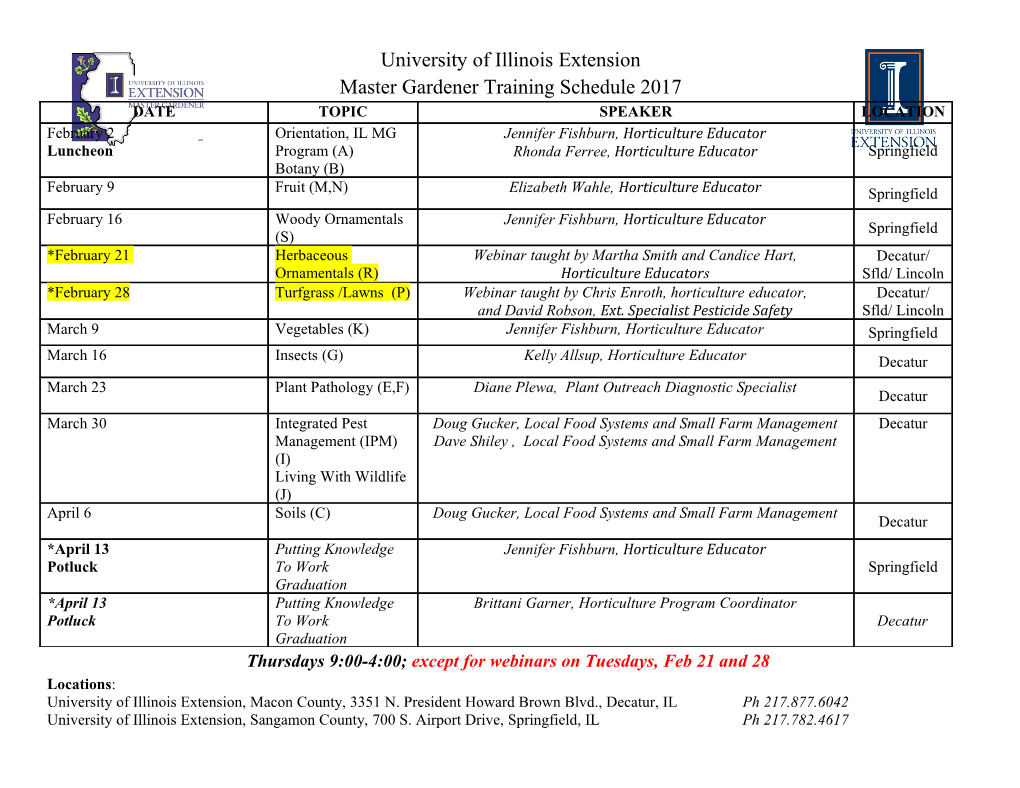
A Molecular Evolutionary Approach for Targeted-transcriptomics of PCB Exposure: A Case Study Involving Hexagenia spp. by Gina Louise Capretta A Thesis presented to The University of Guelph In partial fulfilment of requirements for the degree of Master of Science in Integrative Biology Guelph, Ontario, Canada © Gina Capretta, DecemBer, 2015 ABSTRACT A MOLECULAR EVOLUTIONARY APPROACH FOR TARGETED- TRANSCRIPTOMICS OF PCB EXPOSURE: A CASE STUDY INVOLVING Hexagenia spp. Gina Louise Capretta Advisor: University of Guelph, 2015 Dr. Mehrdad Hajibabaei This thesis developed a methodology to target candidate xenobiotic- interacting genes conserved across taxa, as well as tested the methodology as a tool to indicate chemical exposure and elucidate possible biological effects. Using PCBs as the chemical class, this thesis identified conserved candidate PCB-interacting genes and designed and tested degenerate primers to amplify those genes in divergent species. Using next-generation sequencing technology, this thesis also investigated the targeted-transcriptome response of H. rigida, a common ecotoxicological test species, following exposure of Hexagenia spp. to PCB-52 in a 96-hour water-only test, in which survivorship and bioaccumulation were also measured. Transcript sequences of target genes were generated for H. rigida and successfully annotated. Significant down-regulation of three genes (HSP90AB1, TUBA1C, and ALDH6A1) elucidated the biological processes that may be disrupted. This research shows the potential for linking molecular events to outcomes at higher levels of biological organization, an approach relevant to environmental risk assessment. Dedication To my family iii Acknowledgements There are many people to whom I owe a debt of gratitude; their contributions helped support and encourage me throughout this journey. Thank you, to my advisor, Dr. Mehrdad Hajibabaei, for challenging me to explore new technologies, new methodologies, and new ideas. Thank you for allowing me to travel to numerous conferences and encouraging me to present talks and posters. To all the members of the Hajibabaei lab (both past and present): Nicole, Mike, Steph, Rachel, Ian, and Katie, thanks for always being there to listen to my frustrations and for providing perspective when the going gets tough. To Shannon, lab manager and technician extraordinaire, thank you for helping me with RNA extractions and providing sound advice on lab protocols. A big thank you to Dr. Shadi Shokralla, for helping me through my entire thesis project, for providing both expertise and time, for guiding me through the sequencing and bioinformatics processes. Thank you to NSERC, OGS, the Ministry of Environment and Climate Change (MOECC), and the Department of Integrative Biology for providing funds for my degree. Thank you to my Advisory Committee. Thank you Dr. Glen Van Der Kraak for your advice and the Mus musculus and Danio rerio cDNA samples. Thank you Dr. Paul Sibley for your valuable comments and suggestions during the editing process. Your insight pushed me to do better. Thank you to Trudy Watson-Leung from MOECC, Aquatic Toxicology, Laboratory Services Branch. Thank you for collaborating on this project, for providing the Hexagenia organisms and set up for my PCB exposures, for providing me with tissue bioaccumulation data and water chemistry results, and thank you for your enthusiasm in integrating gene expression analysis in the work you do. Last, but not least, thank you to my family. To my daughters, Anabel and Elsa, who remind me everyday the importance for young girls to have strong and positive female role models in science. Thank you to my mom, dad, and sister for always encouraging my scientific pursuits. Thank you to Joel, my best friend, my colleague, and my partner in life – you encouraged me to start a Master’s, encouraged me to stick with it, and you are still encouraging me as I write this, providing me with your knowledge, expertise, guidance, support, time and above all else, love. iv Table of Contents Chapter 1. General Introduction ……...…………………………….. 1 Chapter 2. In silico identification of candidate PCB-interacting genes conserved in animals Introduction ………………………………………6 Materials and Methods ………………………………………9 Identification of candidate PCB-interacting genes with known invertebrate homologues ………………………………………9 Sequence alignment and analysis …………………………………….10 Functional characterization …………………………………….10 Results …………………………………….11 Discussion and Conclusion …………………………………….12 Tables and Figures …………………………………….16 Chapter 3. Design and validation of degenerate primers for candidate PCB-interacting genes conserved in animals Introduction …………………………………….22 Materials and Methods …………………………………….24 CODEHOP degenerate primers design …………………………………….24 Validation of degenerate CODEHOP primers …………………………………….25 RNA isolation and cDNA synthesis …………………………………….26 PCR amplification …………………………………….27 Visual confirmation …………………………………….28 Bioinformatics confirmation …………………………………….28 Sanger sequencing and analysis …………………………………….28 Results …………………………………….29 Discussion and Conclusion …………………………………….31 Tables and Figures …………………………………….34 Chapter 4. Assesssing the targeted-transcriptome response of Hexagenia rigida to PCB-52 using PCB-interacting genes conserved across taxa Introduction …………………………………….42 Materials and Methods …………………………………….45 Exposures …………………………………….45 Tissue bioaccumulation of PCB-52 …………………………………….46 Selection of candidate genes …………………………………….46 DNA barcoding for species verification …………………………………….47 Total RNA-extraction for targeted- transcriptomics …………………………………….47 RT-PCR for cDNA synthesis …………………………………….48 v Library preparation for Illumina MiSeq sequencing …………………………………….48 Sequencing on Illumina MiSeq …………………………………….49 Data pre-processing …………………………………….49 Annotation and alignment …………………………………….49 Statistical analysis on technical replicates …………………………………….50 Differential gene expression analysis …………………………………….51 Results …………………………………….52 Discussion and Conclusion …………………………………….53 Tables and Figures …………………………………….62 Chapter 5. General Conclusion …………………………………….66 References …………………………………….68 Appendix …………………………………….81 vi List of Tables Table 2.1 Number and sources of mRNA sequences used. 6.23 ± 0.75 = average (± SD) number of sequences per gene alignment 109 = total number of homologous PCB-interacting genes, at least 1 invertebrate sequence/gene; 279.86 ± 116.88 base pairs = average (± SD) length of domain per gene. Table 2.2 Domain conservation of candidate PCB-interacting genes in each KEGG pathway. Table 2.3 Functional characterization of candidate PCB-interacting conserved across taxa (N=109). * = multi-functional ; Lower conservation: < 49.5% conservation/domain; Higher conservation: ≥ 49.5% conservation/domain. Table 3.1 Candidate PCB-interacting genes conserved in animals used for degenerate primer design (N=71 ≥ 49.5% nucleotide conservation for domain selected). Table 3.2 Annealing temperature for each gene’s primer pair. *=housekeeping gene. Primers in bold were tested on Sample 2 of H. limbata. Table 3.3 Number of primer sets considered successful at each confirmation level for each species. Table 4.1 Degenerate primers used in targeted RNA-seq analysis. * housekeeping gene; TA = annealing temperature; Uppercase letters = non degenerate core; Lower letters = degenerate clamp. Insect homologue gene name and symbol is represented by D. melanogaster. Table 4.2 Water chemistry, survivorship, and tissue bioaccumulation results from 96 hour PCB-52 exposure. Table 4.3 Number of each species of Hexagenia per treatment. Table 4.4 Sequencing and annotation results from initial bioinformatics processing. Table 4.5 Log2 fold change values of all genes for the acetone (carrier solution) control and 0.033 µg/L PCB-52. Asterisks denote log2 fold changes ≥ |1| that are statistically significant (p < 0.05). vii Table 4.6 Induction of HSP90 by several environmental stressors in various invertebrate species. Appendix Table A1. Candidate PCB-interacting genes conserved in animals (N=109). Table A2. Summary statistics for domain nucleotide conservation of candidate PCB-interacting genes per KEGG pathway. Table A3. Summary statistics for domain amino acid conservation of candidate PCB-interacting genes per KEGG pathway. Table A4. CODEHOP primers and primer properties (N=68). Upper case letters = non-degenerate core; Lower case letter = degenerate clamp; D=degeneracy; Tm=melting temperature; *=housekeeping gene. Table A5. BLAST output for all genes tested using CODEHOP primers for all species. Q=query; SL=sequence length. List of Figures Figure 1.1 Generic structure of PCBs. Numbering is on the left ring and positioing on the right ring (From Richardson and Schlenk 2011). Figure 1.2 Thesis conceptual framework, divided by objective. A candidate PCB-interacting gene is a gene with a documented effect on gene expression associated with any polychlorinated biphenyl in any organism across any level of biological organization. Figure 2.1 Workflow to identify candidate PCB-interacting genes conserved across taxa using available online databases and bioinformatics tools used in molecular evolution. * nucleotide conservation is represented by the number of identical bases over the total numbers of bases for the region selected. Figure 2.2 Relative frequency of candidate PCB-interacting genes conserved in animals per KEGG Pathway. Figure 3.1 CODEHOP design strategy of degenerate primers for PCB-interacting genes conserved in animals * organisms
Details
-
File Typepdf
-
Upload Time-
-
Content LanguagesEnglish
-
Upload UserAnonymous/Not logged-in
-
File Pages111 Page
-
File Size-