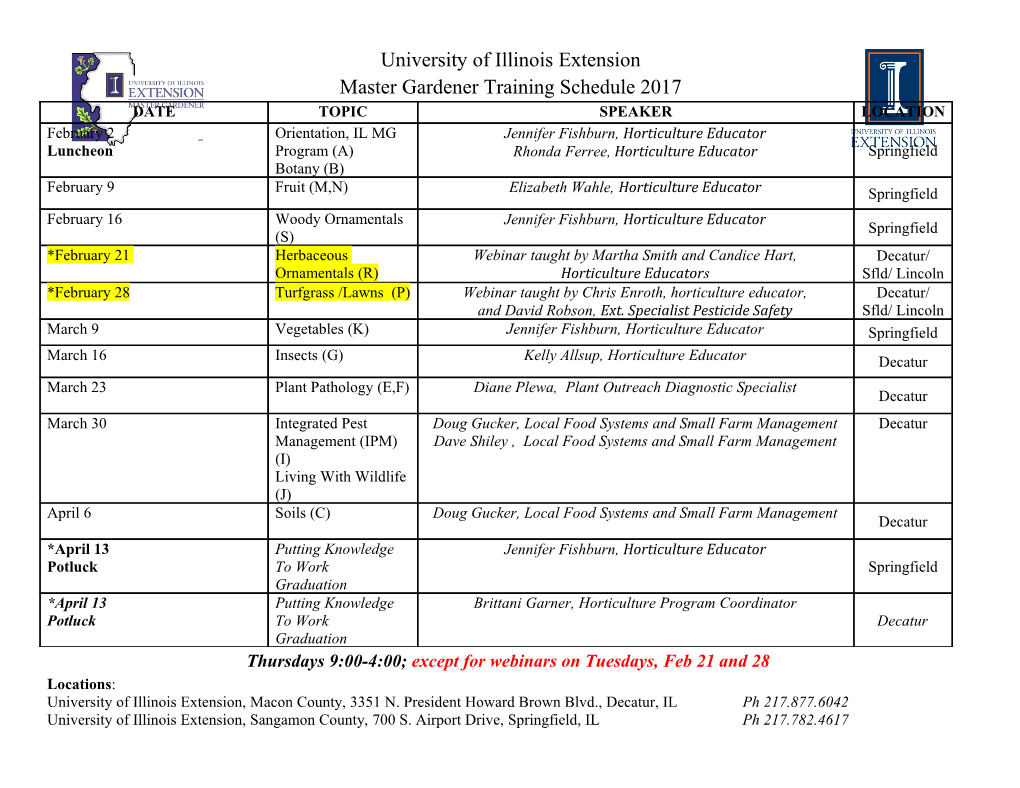
Subsurface of Overburden for Possible Meteorite Impact Crater at Bukit Bunuh, Malaysia using 2-D Electrical Resistivity Imaging Mark Jinmin Postgradute Student, Geophysics Section, School of Physics, 11800 Universiti Sains Malaysia, Penang, Malaysia e-mail: [email protected] Dr. Rosli Saad Senior Lecturer Geophysics Section, School of Physics, 11800 Universiti Sains Malaysia, Penang, Malaysia e-mail:[email protected] Dr. M. Mokhtar Saidin Assoc. Prof., Centre for Global Archeological Research Malaysia, 11800 Universiti Sains Malaysia, Penang, Malaysia e-mail:[email protected] Y.C. Kiu Postgradute Student, Geophysics Section, School of Physics, 11800 Universiti Sains Malaysia, Penang, Malaysia e-mail: [email protected] Nur Azwin Ismail Postgradute Student, Geophysics Section, School of Physics, 11800 Universiti Sains Malaysia, Penang, Malaysia e-mail: [email protected] ABSTRACT Bukit Bunuh is situated in Lengggong, Perak one of Peninsular Malaysia’s most important areas for archeology that revealed many traces of Malaysia’s prehistory. Geophysical method especially 2-D resistivity imaging method is non-destructive which applied in geo-subsurface study for meteorite impact. The study consists of three stages that are regional study and detail study. Total of fourteen survey lines, which include parallel to Sungai Perak and two mountains ranges, Bintang Range and Titiwangsa Range. Survey lines were executed using Pole-dipole array with 5 m minimum electrode spacing. From every stage, the results show the area consists of two main layers which are alluvium and granitic bedrock. The first zone is alluvium with boulders with resistivity value of 10-800 Ωm while the second zone is granitic bedrock with resistivity value of >1500 Ωm. This study also shows few spotted possibility of uplift (rebound) due to the impact, a lot of fractures were found along the survey lines which could be one of the effect of meteorite impact. KEYWORDS: Bukit Bunuh, 2-D resistivity electrical imaging, overburden, regional, detail, rebound. - 1533 - Vol. 19 [2014], Bund. G 1534 INTRODUCTION Impact cratering plays a major role in surface forming and modifying of planetary bodies. It is also rarely considered by the most geoscientist due to other dynamic endogenic processes and yet it is a very rare event by the human time scale. (Philip, 2004), It is recognized as one of the fundamental processes in the geological evolution of the Earth. Studies on terrestrial impact craters can give necessary information about planetary evolution and influence the terrestrial environment, including the biosphere. (Tiiu, 2011) Hypervelocity impact that collide with the earth surface will the structural and mineralogical changes in the target will also affect the petrological properties of target rocks up to few kilometers or even more. During penetration stage the projectile collides with the target and enormous amount of kinetic energy is released and transformed into high pressures and temperatures. The material affected by the impact is compressed and pushed outward from the point of impact while the shock waves travel away and lose the energy due to reduction of the overall energy density with increasing area. The target rock is deformed and heated Gravity method can detect the contrasts beneath the surface which will provide constraints on the geometry of the crater. Impact craters can be classified as simple or complex based on their size and morphology (French, 1998). From Pilkington and Grieve (1992) and Grieve and Pilkington (1996), the authors suggest that a negative gravity anomaly is most common signature coinciding with impact craters. The density contrast may due to the fractured rock filled with under dense fragmental breccias and sediments is less dense the unaltered rock surround and exhibit a generally circular structural boundaries. Negative gravity anomaly is not a definite sign of impact. In complex impact structures, where subcrater fracturing and brecciation are accompanied by uplift of denser deep- seated rocks into the central part of the structure, the normal negative gravity anomaly may be reduced or even converted to a positive anomaly, because the uplifted denser rocks overcome the effects of fracturing and brecciation (Stepto, 1990; Pilkington and Grieve, 1992; Grieve and Pilkington, 1996). Site observation indicates the presence of suevite rock around Bukit Bunuh in various size and shape (Nawawi, 2009). In the center of the survey area, a lot of cobbles and pebbles(Figure 1) found which identified as impacted rocks. Shattering and brecciation of the rocks that already exist on and beneath the crater floor detected is one of the evidence of meteorite impact at Bukit Bunuh, Lenggong which is a northern state of Perak. In this investigation of impact crater studies, the non invasive of gravity geophysical method are conducted and illustrated with case studies subsurface interfaces and boundaries such as a stratigraphy boundary or some physical discontinuity (faults/fractures) based on density body. Vol. 19 [2014], Bund. G 1535 A B Figure 1: Schematic cross-section of simple crater shown in A and central peak impact crater shown in B (after Grieve, 1987). 2-D RESISTIVITY IMAGING METHOD Theory stated that direct-current resistivity method is used to determine the electrical resistivity structure of the subsurface (Figure 2 and 3). Resistivity is defined as a measure of the opposition to the flow of electric current in a material. The resistivity of a soil or rock is dependent on several factors that include amount of interconnected pore water, porosity, amount of total dissolved solid such as salts and minerals compositions (clay). The 2-D DC resistivity method is described by Zohdy and others (1974), Summer (1976), Reynolds (1997) and Rubin and Hubbard (2006). The resistivity method basically measures the resistivity distribution of the subsurface materials. Table 1 shows the resistivity value of some typical rocks and soil materials (Keller and Frischknecht, 1996). Vol. 19 [2014], Bund. G 1536 Table 1: Resistivity values of common rocks and soil materials (Keller and Frischknecht, 1996). Material Resistivity (ohm-m) Alluvium 10 to 800 Sand 60 to 1000 Clay 1 to 1000 Groundwater (fresh) 10 to 100 Sandstone 8 - 4 x 105 Shale 20 - 2 x 105 Limestone 50 - 4 x 105 Granite 5000 to 1,000,000 Figure 2: Four-point electrode configuration in a two-layer model of resistivity, ρ1 and ρ2. I, current; U, voltage; C, current electrode; P, potential electrode (Said, A,H., 2007). Figure 3: Acquisition of a 2D apparent resistivity pseudosection using dipole–dipole array (C1 C2 P1 P2). C, current electrode; P, potential electrode; a, dipole spacing; n, dipole factor (Said, A,H., 2007). Vol. 19 [2014], Bund. G 1537 STUDY AREA This study was carried out around the area of Bukit Bunuh, Lenggong, Perak (Malaysia). The area of the surveys covered 132.25 km2 from from Lenggong Town, Perak (Figure 4). Data are acquired with respect to the accessible of the study area. The study was consisted of regional study (Stage 1) and detail study (Stage 2 and Stage 3). In regional study, total of four resistivity survey lines whereas in detail study total of ten resistivity survey lines were executed. The topography of Bukit Bunuh is 600 feet’s above MSL. Perak River is situated on the east of Bukit Bunuh which flows from north to south at the elevation of 61 feet’s MSL. There are borehole records in line with survey lines. Figure 4: Topography of study area at Bukit Bunuh with 2-D Electrical survey lines. Vol. 19 [2014], Bund. G 1538 METHODOLOGY The 2-D electrical resistivity imaging survey was conducted using ABEM SAS4000 Terrameter, ES10-64C as a selector, electrode cables with minimum 5 m spacing and stainless steel electrodes. The pole-dipole array was used with roll-along technique. 2-D resistivity data was modeled using Res2Dinv software. The data then outputted into Res2Dinv and Surfer software for gridding and contouring. RESULTS AND DISCUSSION Complete with data acquisition and data processing which are very crucial process in geophysical method, 2-D resistivity results generally show the area was divided into two main zones. The first zone with resistivity value of 10-800 ohm and thickness approximately 5-60 m was interpreted as alluvium. There are boulders with resistivity value of >6000 ohmm which embedded in the alluvium. The second zone with resistivity value >2000 ohmm was bedrock. The resistivity results were match with borehole record (Figure 5 and 6). Lots of fractures were found along the survey line which due to the meteorite impact. Figure 7 shows 2D view of bedrock (overburden) at the study area with suspected rebound (R) on the map. Figure 5: Resistivity pseudo-section Line 2 (0-800 m) with borehole record at 185 m (Detail Study) Vol. 19 [2014], Bund. G 1539 2 silt with some gravel - very stiff 4 silt with some gravel - very stiff 6 silty gravel - reddish ligh 8 sandy gravel - medium de 10 - light grey gravel yellowish dark brown 12 14 silt with some gravel - very stiff 16 - hard sandy gravel 18 - No Recovery 20 22 sandy gravel - light grey y - No Recovery Figure 6: Borehole record at resistivity line 2 at 185 m (BH2). Vol. 19 [2014], Bund. G 1540 100.96 100.98 101 101.02 5.14 5.14 MSL Height (m) 5.12 5.12 280 260 240 5.1 5.1 220 200 180 160 5.08 5.08 R R 140 R 120 R 100 5.06 5.06 80 70 60 40 5.04 5.04 20 0 -20 -40 5.02 5.02 -60 Bedrock 5 5 100.96 100.98 101 101.02 Figure 7: 2-D view of overburden in study area. CONCLUSION The present study shows that the 2-D resistivity electrical imaging on contouring the variations of subsurface features at impact structure area is a useful approach.
Details
-
File Typepdf
-
Upload Time-
-
Content LanguagesEnglish
-
Upload UserAnonymous/Not logged-in
-
File Pages11 Page
-
File Size-