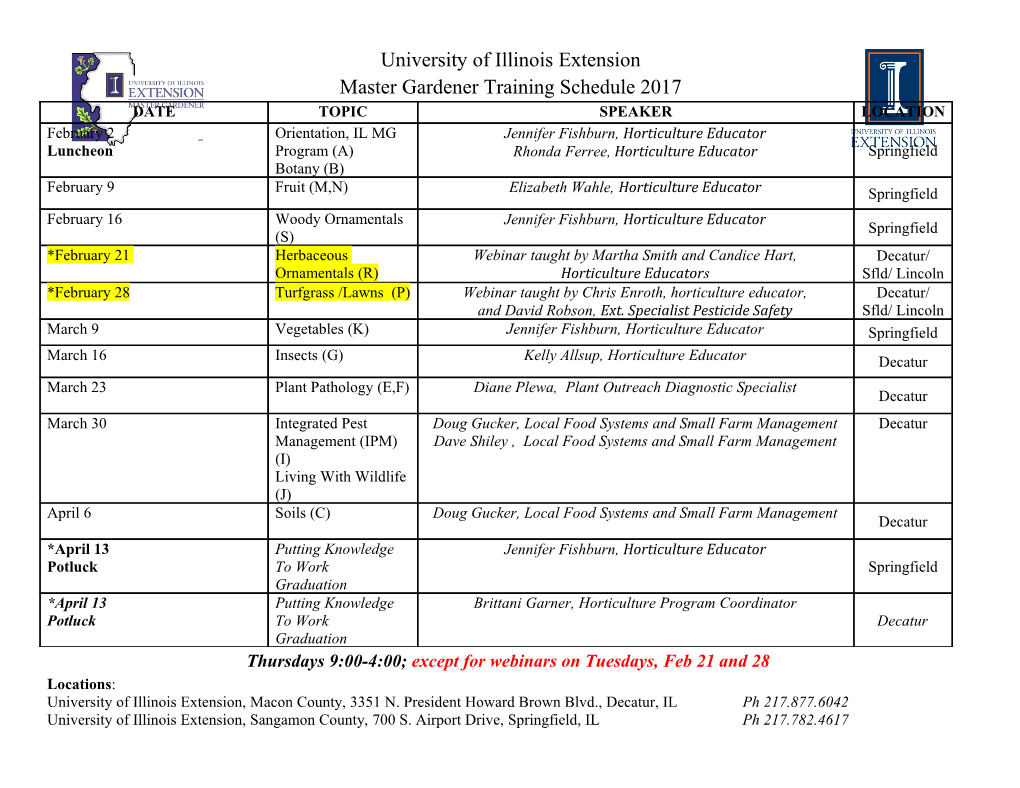
Britton Smith Institute for Astronomy University of Edinburgh Sadegh Khochfar Mike Norman Brian O’Shea John Wise Where did the first Pop II stars come from? Aspen March 9, 2016 The Astrophysical Journal,781:60(22pp),2014February1 Hirano et al. Table 2 Evolutionary Paths 105 106 Mvirial [M ] Path M N ⊙ sample ˙ 1 (M yr− ) 18 ⊙ 16 P1 KH Contracting protostar <0.004 67 P2 Oscillating protostar >0.0041 31 14 P3 Supergiant protostar >0.042 12 12 10 Notes. Column 2: accretion rate for each path, and Column 3: the halo N 8 number of stars in our sample. References. (1) Omukai & Palla 2003; (2) Hosokawa et al. 2012a. 6 4 2 represented by white circles corresponding to 1 pc. As expected, 0 the five clouds have different structures of density, velocity, and 40 35 30 25 20 15 10 temperature. The resulting stellar masses are also different as z form indicated in the figure. Figure 2. Number of dark matter halos that host star-forming gas clouds. After the formation of a protostellar core at the center of The histogram shows the distribution of redshifts when the central gas density the collapsing cloud, we switch to the 2D RHD calculations 6 3 reaches 10 cm− . The histograms are colored according to the virial masses for each individual dark matter halo and follow the evolution using the∼ color scale displayed at the top. during the later accretion stages. Figure 4 shows snapshots from (A color version of this figure is available in the online journal.) three of our examined cases, which exemplify the three different evolutionary paths (P1, P2, and P3). We see that in each case SPH cosmological simulations to follow the formation of the abipolarHii region forms (Figure 4(a)), which subsequently primordial gas clouds that gravitationally collapse in the centerPopgrows at varying rates as the stellarIII mass increases. The mass to Pop II of dark matter halos. The histogram in Figure 2 shows that our accretion onto the protostar is finally shut off by the strong UV sample of 110 dark matter halos has a wide range of masses radiative feedback caused by the dynamical expansion of the Hii 5 6 Mvirial 10 –10 M distributed over redshifts z 35–11, region (Figure 4(c); see also Hosokawa et al. 2011). Figure 5 most of= which are at z⊙ 20–15. Figure 3 shows an example= of shows the distribution of the final stellar masses obtained in the resulting gas density= concentrations arising in five such dark our simulations (a summary is given in Table 2). We see a matter halos together with insets of their zoomed-in structure, large scatter of resulting stellar masses, ranging from 9.9 M Transition⊙ Pop III Figure 3. Projected gas density distribution at z 25 in one of our cosmological simulations. We show five primordial star-forming clouds in a cube of 15 kpc on a side. The circles show the zoom-in to the central= 1 pc region of theHirano clouds at the respective ea. formation epoch.(2014) The masses of the first stars formed in these clouds are ? 60, 76, 125, 303, and 343 M ,respectively. ⊙ (A color version of this figure is available in the online journal.) 5 Matt Turk ‣ Pop III star formation seems fundamentally distinct. ‣ Pop III to Pop II transition informs the nature of stellar IMF. ‣ Metal-free stars have distinct spectral properties. Pop II,… ‣ Transition era (z ~ 10–20) starting to be probed. What is the stellar content of these galaxies? The First Low-Mass Stars 630 OMUKAI ET AL. Vol. 626 ‣ metals add cooling, decrease Jeans mass ‣ fragmentation is a 3D problem, AND highly dependent on initial conditions Fig. 1.—Temperature evolution of prestellar clouds with different metallicities. Those with metallicities Z/H (Z 0), 5, 3, and 1( 6, 4, 2, and 0) are shown by solid (dashed) lines. Only the present-day CMB is considered as an external radiationOmukai field. The½ lines ¼À forea.1 constant¼ Jeans(2005)À massÀ areÀ indicateÀ dÀ by thinÀ dotted lines. The positions at which the central part of the clouds becomes optically thick to continuum self-absorption is indicated by the thin solid line (eq. [20]). The intersection of the thin solid line with each evolutionary trajectory corresponds to the epoch when the cloud becomes optically thick to the continuum. To the right of this line, the clouds are optically thick and there is little radiative cooling. [See the electronic edition of the Journal for a color version of this figure.] Omukai (2000) for a thorough discussion of this issue. The initial In Figure 2 we illustrate separately the contribution by each ionization degree and H2 concentration are assumed to be the processes to cooling/heating rates in the fiducial cases. For the values for uniform matter in the postrecombination universe: same cases, we also show the evolution of H2 and HD fractions 4 6 y(e) 10À , y(H2) 10À .Initially,carbonisfullyionized in Figures 3 and 4, respectively. In terms of major coolants, the and helium¼ and oxygen¼ are neutral. thermal evolution can be classified into the following three metallicity ranges: (1) quasi-primordial clouds ( Z/H P 6), 3. RESULTS (2) metal-deficient clouds ( 5 P Z/H P 3), and½ (3) metal-À enriched clouds ( 2 P Z/HÀ). For½ each of theseÀ ranges, we now In this section, we first present the thermal and chemical evo- À ½ lution for different initial values of the gas metallicity ( 3.1); describe the evolution presented in Figures 1–4. then, a reduced chemical model for low-metallicity cloudsx is 1. Quasi-primordial clouds ( Z/H P 6).—The presence of metals at metallicity levels as low½ as [Z/HÀ P 6doesnotsig- presented ( 3.2). Using the derived effective equation of state, we À discuss thex fragmentation properties of prestellar clouds and es- nificantly alter the thermal evolution in any density range. The timate the typical mass scales of fragmentation in the Appendix. evolution of both temperature and chemical species follows closely those of the metal-free case. Molecular hydrogen is always an 3.1. Thermal and Chemical Evolution important cooling agent. HD hardly affects the overall evolution 5 3 3.1.1. Results for the Fiducial Cases despite contributing as much to the cooling as H2 at 10 cmÀ (see also Bromm et al. 2002). The temperature evolution for prestellar clouds for different To clarify the reasons for this trend, we first describe the metallicities is shown in Figure 1. The cases with metallicities HD formation process. The abundance of HD is determined by Z/H (i.e., Z 0 metal-free case), 5, 3, and 1 ½ ¼À1 ¼ À À À the equilibrium between the formation reaction (reaction D4 in ( Z/H 6, 4, 2, and 0) are indicated by solid (dashed) Table 1) and its inverse dissociation reaction (D6), lines.½ As¼À an externalÀ À radiation field, only the present-day (2.73 K) CMB is included, although its effect can be neglected except for D4; D6: Dþ H2 Hþ HD: 12 in the lowermost temperature regime in the Z/H 0case.We þ $ þ ð Þ ½ ¼ refer to this set of models as the fiducial cases hereafter. The The deuterium ionization degree is set by the equilibrium be- dotted lines indicate those of a constant Jeans mass. The dashed tween reactions D1 and D2, line labeled ‘‘J 1’’shows the location where the central part of a cloud becomes¼ optically thick to continuum radiation (eq. [20]; D1; D2: D Hþ Dþ H: 13 see discussion below). Before this condition is met, i.e., to the left þ $ þ ð Þ of the line, clouds are still optically thin to the continuum. The HD to H ratio is then The evolution of clouds with metallicity Z/H (4 3) at 2 4 8 3 ½ ¼À nH 10 À cmÀ is mostly affected by the improvements of the n(HD) k n(D ) k k n(D) 421 K n(D) D4 þ D4 D1 2exp ; model, namely, by the inclusion of HD cooling and the modifi- n(H ) ¼ k n(H ) ¼ k k n(H) ¼ T n(H) cation of the collapse timescale. Conversely, both at lower and 2 D6 þ D6 D2 14 higher metallicity values, the thermal evolution is hardly altered. ð Þ The First Low-Mass Stars 630 OMUKAI ET AL. Vol. 626 ‣ metals add cooling, decrease Jeans mass gas metals ‣ fragmentation is a dust 3D problem, AND highly dependent on initial conditions Fig. 1.—Temperature evolution of prestellar clouds with different metallicities. Those with metallicities Z/H (Z 0), 5, 3, and 1( 6, 4, 2, and 0) are shown by solid (dashed) lines. Only the present-day CMB is considered as an external radiationOmukai field. The½ lines ¼À forea.1 constant¼ Jeans(2005)À massÀ areÀ indicateÀ dÀ by thinÀ dotted lines. The positions at which the central part of the clouds becomes optically thick to continuum self-absorption is indicated by the thin solid line (eq. [20]). The intersection of the thin solid line with each evolutionary trajectory corresponds to the epoch when the cloud becomes optically thick to the continuum. To the right of this line, the clouds are optically thick and there is little radiative cooling. [See the electronic edition of the Journal for a color version of this figure.] Omukai (2000) for a thorough discussion of this issue. The initial In Figure 2 we illustrate separately the contribution by each ionization degree and H2 concentration are assumed to be the processes to cooling/heating rates in the fiducial cases.
Details
-
File Typepdf
-
Upload Time-
-
Content LanguagesEnglish
-
Upload UserAnonymous/Not logged-in
-
File Pages25 Page
-
File Size-