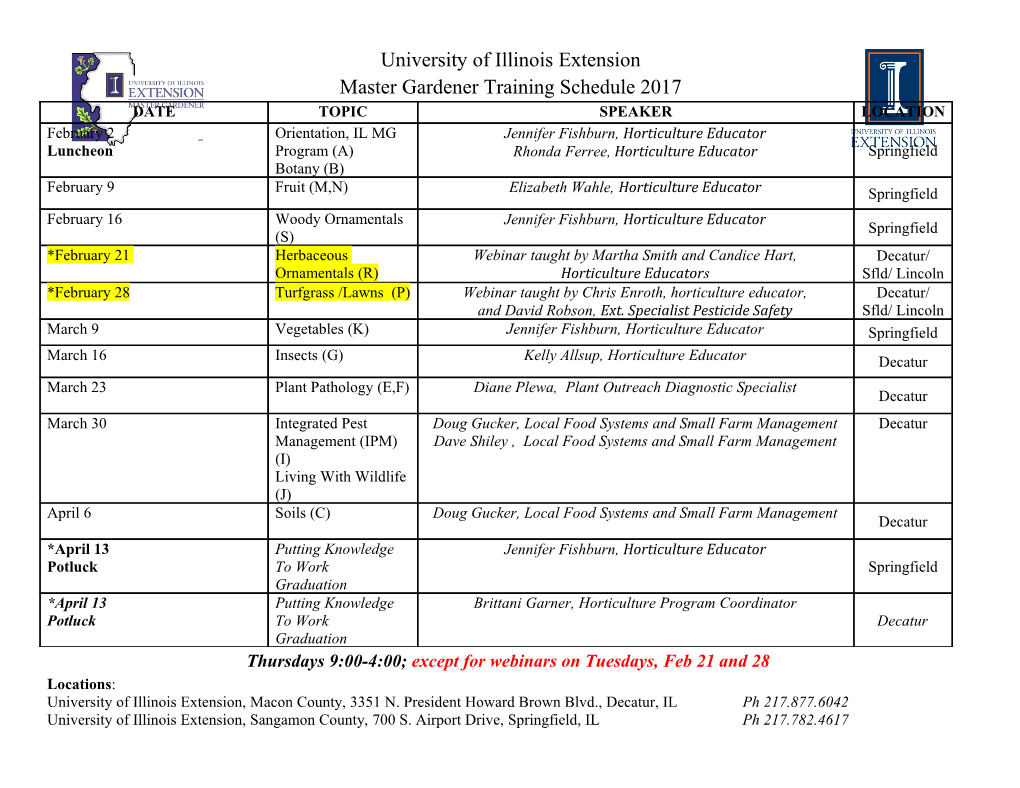
1 Wind Sea and Swell Waves in the Nordic Seas Alvaro Semedo1,2 Escola Naval-CINAV, Lisbon, Portugal. Roberto Vettor Centre for Marine Technology and Engineering (CENTEC), Instituto Superior Técnico, University of Lisbon, Lisbon, Portugal. Øyvind Breivik European Centre for Medium-Range Weather Forecasts, Reading, United Kingdom Andreas Sterl Royal Netherlands Meteorological Institute (KNMI), De Bilt, Netherlands Magnar Reistad Norwegian Meteorological Institute, Oslo, Norway Carlos Guedes Soares Centre for Marine Technology and Engineering (CENTEC), Instituto Superior Técnico, University of Lisbon, Lisbon, Portugal. 1. Introduction The waves at the ocean surface are the most obvious air-sea interaction phenomena at the interface between the atmosphere and the ocean. These wind waves (henceforth simple called waves) account for most of the energy carried by all waves at the ocean surface (Kinsman 1965), and have a significant impact on coastal infrastructures, ship design and routing, coastal erosion and sediment transport, and are an important element in storm surges and flooding events. Two types of ocean waves can be identified at the ocean surface: wind sea and swell. Wind sea waves are waves under the influence of local winds. As waves propagate from their generation area, or when their phase speed is higher than the local wind speed, they are called swell. Swell waves can propagate thousands of kilometers across entire ocean basins (Snodgrass et al. 1966; Alves 2006). The significant wave height (SWH) and the mean wave period (MWP) are the most common parameters used to characterize sea states. But these parameters provide a limited description of the wave field, since they are calculated by integrating the wave 1 Corresponding author address: Alvaro Semedo, Escola Naval-CINAV, Base Naval de Lisboa, Alfeite, 2810-001 Almada, Portugal. E-mail: [email protected] 2 Also affiliated with: Centre for Marine Technology and Engineering (CENTEC), Instituto Superior Técnico, University of Lisbon, Lisbon, Portugal, and to Department of Earth Sciences, Uppsala University, Sweden. 2 spectra, and two wave fields with the same SWH and MWP may still be different in detail. A mixed sea state of wind sea and swell waves can have the same SWH and MWP as a more young sea state with a strong prevalence of wind seas almost without swell (Holthuijsen 2007, Semedo et al. 2011). For this reason a more detailed investigation and qualitative analysis of the wave field is needed to correctly define the wave characteristics of a certain area. The way to pursuit this analysis is by studying the wind sea and swell parameters separately [SWH and MWP, but also mean wave direction (MWD)]. Several recent studies have looked at the qualitative separation of ocean surface waves has been the focus of several recent climatological studies (Chen et al. 2002, Gulev and Gregorieva 2006, Semedo et al. 2011, Jiang and Chen 2013). Some other studies, following an air sea interaction perspective (Smedman et al. 2009, Högström et al. 2009, Semedo et al. 2009) have also looked at the wind sea and swell separation of the ocean surface. The reason for this interest from the air-sea interaction community lies mostly on the fact that waves have an impact on the lower atmosphere, and that the air-sea coupling is different depending on the wave regime. Waves modulate the exchange of momentum, heat, and mass across the air-sea interface and this modulation is different, depending on the prevalence of one type of waves: wind sea or swell (Högstrom et al. 2009, Semedo et al. 2009). The goal of the present study is to present a climatology of wind sea and swell characteristics in the Nordic Seas (North Sea, Norwegian Sea, and Barents Sea), based on the high resolution Norwegian Reanalysis at 10 km (NORA10; Reistad et al. 2011), following a similar methodology as Semedo et al (2011). The NORA 10 is a dynamically downscaled version of the ERA-40 reanalysis (Uppala et al. 2005), which comprises atmospheric and wave parameters. The regional distribution of the wind sea and swell SWH and MWD parameters from NORA10, and how they combine in the total SWH and MWP, are presented. 2. Data and analysis methodology a. NORA10 The NORA 10 is a 3-hourly atmospheric and wave high resolution regional reanalysis, produced with the dynamic downscaling of the European Centre for Medium-Range Weather Forecasts (ECMWF) ERA-40 reanalysis. The ERA-40 covers a period from September 1957 to August 2002. Although the NORA 10 is continuously being extended after 2002 using operation analyses from the ECMWF as boundary and initial conditions, here we use only the NORA10 period coincident with ERA-40 (1958- 2001), for homogeneity purposes but also to allow some degree of comparison with the results in Semedo et al. (2011). The NORA10 atmospheric reanalysis was produced with the hydrostatic High- Resolution Limited Area Model (HIRLAM; Undén et al. 2002) at 10-11 km resolution (HIRLAM10), with 40 vertical levels, denser at the surface. The NORA10 model domain was set up as a rotated spherical grid, with a false South Pole (Reistad et al. 2011, their Figure 1). The wave component of the NORA10 was produced by forcing the wave model WAM (cycle 4; WAMDI Group 1988; Komen et al. 1994) with HIRLAM10 winds on the same domain as the atmospheric model domain. An inner wave model domain was nested into a larger 50 km resolution domain that generated swell boundary conditions. The outer domain was forced with ERA-40 winds. Ice coverage information was passed 3 to the wave model from HIRLAM10 three times per month. Both outer and inner domains were integrated with the bottom friction mode on. Further details and results on NORA 10 can be seen in Reistad et al. (2011) and Aarnes (2011). b. Wave parameters and spectral separation The wave model WAM outputs two-dimensional wave energy spectra ( , ) at each grid point by integrating the so called wave energy balance equation, where and are the wave frequency and propagating direction, respectively. From 퐹the푓 wave휃 energy spectra several integrated wave parameters can be obtained. Here SWH,푓 and MWD휃 (mean wave direction) from the total ( , ), wind sea ( , ) and swell ( , ) wave fields, respectively, are used. Besides the wave fields 푤the 푤NORA10 10 푠 푚 푠 푚 m wind푠 푠 speed ( ) and direction ( ) are also 퐻used.휃 The SWH (Munk퐻 1944)휃 is defined 푠 푚 as퐻 휃 = = 4.04 , where is the zeroth spectral moment defined as 푈10 휑 푠 0 0 푆푊퐻 =퐻 (�,푚) , 푚 (1) 0 0 and the 푚MWD∬ is푓 defined퐹 푓 휃as푑푓푑휃 = atan ( ), where 푚 = sin ( ) ( ,휃 ) and푆퐹 ⁄ 퐶퐹 (2a) 푆퐹 = ∬ cos (휃 )퐹 (푓 ,휃 )푑푓푑휃 . (2b) In퐶퐹 the ∬wave model휃 퐹 푓 WAM휃 푑푓푑휃 the wind sea and swell parameters are obtained by integrating the high and low frequency parts of the wave spectra, respectively. The spectral partitioning is obtained through a separation frequency , with a correspondent wave phase speed defined as ̂ 푓 = 33.6 × cos ( ) (3) ∗ where 푐̂ is the friction푢 velocity.휃 − 휑 See Bidlot (2001) and Semedo et al (2011) for further details on the wind sea and swell separation scheme. ∗ 푢 3. The Nordic Seas wind sea and swell climates The wind and wave parameters were processed for seasonal means: December- January-February (DJF), March-April-May (MAM), June-July-August (JJA), and September-October-November (SON). In spite of some mentions in the text to the intermediate seasons MAM and SON, we will only look at the DJF and JJA seasons (Boreal winter and summer, respectively). Figure 1 shows the seasonal maps of the DJF and JJA and (arrows) climatological means are shown. During the winter the mean 10 m-wind speed is higher 10 than 11 m.s-1 in almost all the Nordic Seas, including in the North 푈Sea, and higher휑 than 12 m.s-1 in an area south of Iceland. In the summer the climatological mean is lower (less than 7 m.s-1 in most of the area), with a maximum (around 9 m.s-1) also south of 10 Iceland. The Norwegian Sea is the preferred track of the extratropical storms푈 in the North Atlantic (Chang et al. 2002; Bengtsson et al. 2006), as can be seen in the southern areas of the domain. South of Iceland the 10 m-winds blow from southwest, as part of 4 the North Atlantic Westerlies, slightly turning counter-clockwise as they enter the Norwegian Sea, both in DJF and JJA. The convergence of the Westerlies with the Polar Easterlies can be seen in both seasons, taking place slightly farther North in JJA. Mesoscale features can be seen in the Barents Sea in both seasons, and along the a) b) Figure 1 – Seasonal averages of U ( ) and (°) for (a) DJF and (b) JJA. The arrows are not scaled with the background fields. −1 10 푚 푠 휑 western coast of Norway in JJA (most probably sea breezes). A slight increase in the in the Nordic Seas in DJF and JJA is noticeable when compared to ERA-40 (see Semedo et al 2011), as expected from the validation of the NORA10 shown in Reistad 10 et푈 al. (2011). The seasonal maps of the , , and (as well as of , , and , represented as arrows) climatological means푠 for DJF푤 and JJA can be seen 푤in Figure 푠2. 푠 푠 푠 푚 푚 푚 While in the open ocean is considerably퐻 퐻 higher퐻 than , as shown 휃by Semedo휃 et휃 al. (2011) and Jiang and Chen푠 (2013), that is not necessarily푤 the case in smaller and 푠 푠 constrained areas like marginal퐻 seas, where the fetches퐻 are limited and swell waves might not have space to propagate away from their generation area.
Details
-
File Typepdf
-
Upload Time-
-
Content LanguagesEnglish
-
Upload UserAnonymous/Not logged-in
-
File Pages14 Page
-
File Size-