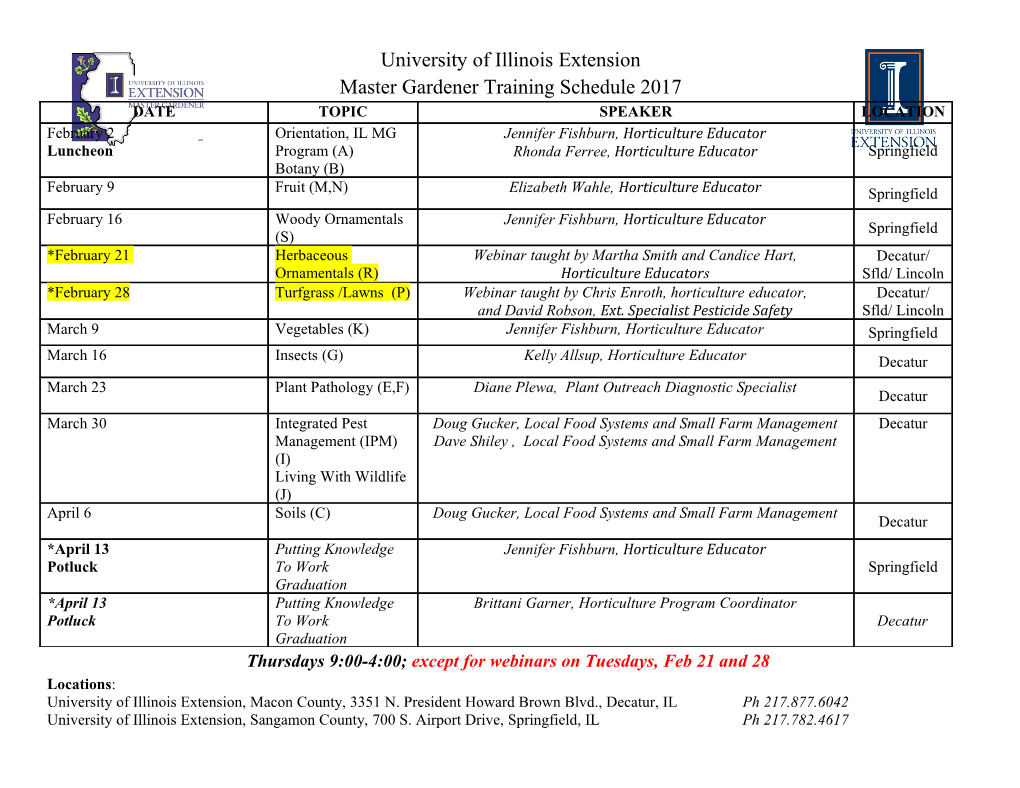
Journal of Mineralogical and Petrological Sciences, J–STAGE Advance Publication, May 22, 2018 Temperature–induced phase transition of AlPO4–moganite studied by in–situ Raman spectroscopy Masami KANZAKI Institute for Planetary Materials, Okayama University, 827 Yamada, Misasa, Tottori 682–0193, Japan The moganite–form of AlPO4 has recently been discovered from our high–pressure study. Similar to SiO2– moganite, a temperature–induced displacive phase transition is expected. In order to confirm the phase transi- tion, high–temperature in–situ Raman spectroscopy study was conducted at ambient pressure up to 600 °C. One of the low–frequency Raman modes (74 cm−1 at room temperature) significantly softened with temperature, and disappeared at 420 °C. Its frequency versus temperature relation can be well fitted with an order parameter equation, and the mode is interpreted as a soft mode with a critical exponent of 0.232(8). According to this fitting, the transition temperature is determined as 415 °C. Some hard modes also revealed slight softening or hardening with temperature up to ~ 420 °C and reached nearly constant frequency at higher temperature. Vibrational mode calculations by the first–principles density functional theory (DFT) method showed that the soft mode corresponds to tetrahedral rotations, representing the pathway of the transformation. Keywords: AlPO4, Moganite, Raman spectroscopy, Phase transition, Soft mode INTRODUCTION of moganite, quartz and amorphous silica. Although there are several reports on synthesis of SiO2–moganite (Schäf Recently, a moganite–type AlPO4 phase was discovered et al., 2006; Kyono et al., 2017), these synthetic samples from high–pressure experiments, and its crystal structure also contain coexisting quartz. Since many Raman peaks was reported (Kanzaki and Xue, 2012). Moganite (SiO2) of moganite overlap with those of quartz as a result of is a metastable phase (Heaney, 1994), whereas AlPO4– their structural similarity (Kingma and Hemley, 1994), it moganite is one of the stable high–pressure polymorphs is difficult to study natural SiO2–moganite by Raman of AlPO4. The structure of moganite is closely related to spectroscopy. Heaney et al. (2007) used a single peak that of quartz and can be regarded as a periodic twinning (at 501 cm−1) for their hard mode Raman study, which according to the Brazil law on a unit–cell dimensional is intense and well separated from other Raman peaks scale (Miehe and Graetsch, 1992). Since temperature–in- of coexisting quartz. They revealed anomalous behavior duced displacive phase transitions are common for the across the transition for this phase. However, it is not low–pressure phases of SiO2 (cristobalite, tridymite, and clear whether this behavior is intrinsic to the phase tran- quartz) and AlPO4, a similar phase transition is expected sition or due to the nature of natural samples with com- for moganite–like phases as well. Using natural SiO2–mo- plicated nanostructures. Also, a high background at lower ganite, Heaney and Post (2001) observed an α–β (I2/a to angle was observed for natural samples in both X–ray and Imab) phase transition at about 570 K using synchrotron neutron diffraction patterns (Heaney and Post, 2001). The X–ray powder diffraction. Heaney et al. (2007) further situation is far better for AlPO4–moganite, for which a studied this transition using hard mode Raman spectros- pure synthetic phase is readily available (Kanzaki and copy, and confirmed the phase transition. A soft mode is Xue, 2012). Although AlPO4–moganite is not stable at expected for this transition, but it has not been observed. ambient pressure, our preliminary heat treatment study Good–quality SiO2–moganite samples are difficult at ambient pressure revealed that it can be heated up to to obtain, and natural samples are often intimate mixtures 600 °C without transforming back to the stable phase (berlinite) at ambient pressure. Therefore, AlPO4–mogan- – doi:10.2465/jmps.171219Advance Publicationite could be used as an analogueArticle of SiO2 moganite for a M. Kanzaki, mkanzaki@okayama–u.ac.jp Corresponding author better understanding of the transition. Although their be- 2 M. Kanzaki haviors may not be identical, it should provide further home–built micro–Raman spectrometer with a 488 nm insight into the phase transition, such as identifying the single–longitudinal–mode solid laser (Coherent Sapphire soft mode and comparing the anomalous behavior ob- SF). It was noted that the frequency of the laser slightly served for SiO2–moganite. depends on the operating power, so a fixed laser power In order to avoid confusion, the room–temper- (80 mW) was used for a series of in–situ runs, as well as ature phase of AlPO4–moganite will be designated as α– during the wavenumber calibration. The laser beam was AlPO4–moganite, whereas the high–temperature phase focused onto a fragment of the sintered sample with a will be designated as β–AlPO4–moganite, where the dis- long working distance 20× objective lens (Mitsutoyo M tinction is necessary. Plan NIR), forming about 1 μm laser spot. Backscattered Al and P replace Si in the SiO2–moganite structure in light was then focused to a pinhole (diameter of 200 μm). an ordered manner to form AlPO4–moganite, and the The passed light was collimated, and passed through two space group of α–AlPO4–moganite (P2/a)isdifferent Ondax’s SureBlock Raman notch filters (Lebedkin et al., from that of α–SiO2–moganite (I2/a) (Kanzaki and Xue, 2011) to reject Rayleigh scattering, and was focused to an 2012). It was also suggested that the expected β–form entrance slit of the polychromator (Acton Spectra Pro would have space group Pmaa (Kanzaki and Xue, 500i with focal length of 500 mm, and a grating of 1200 2012). However, no crystal structural refinement of β– gr/mm). The dispersed light was detected by a liquid AlPO4–moganite has been conducted to date. In this N2 cooled CCD detector (Princeton Instruments, PyLoN study, the phase transition of α–AlPO4–moganite was ex- 100BR eXcelon) with temperature set at −120 °C. The plored by high–temperature in–situ micro–Raman spec- present setup can measure Raman spectra down to ~ 15 −1 troscopy. Although no soft mode was observed in SiO2– cm including the anti–Stokes side. moganite by Heaney et al. (2007), they suggested the pos- For in–situ high–temperature Raman study, a wire– sibility that a soft mode is hidden in the low–frequency heater cell reported in our previous study (Kanzaki et al., region, as their measurement below 100 cm−1 was highly 2012) was used. For heating, a digital DC power source attenuated by the Raman notch filter they used. In this (Kenwood PU8–90) was used. Temperature was calibrat- study, newly available volume notch filters were em- ed against heating power of the DC power supply using ployed to explore the low–frequency region down to the melting points (Tm) of the following materials: −1 ~15cm , where the soft mode is expected. NH4NO3 (170 °C), NaNO3 (308 °C), CsNO3 (414 °C), LiCl (605 °C), and NaCl (801 °C). The melting points of EXPERIMENTAL PROCEDURES these materials were determined by visual observation under the microscope of the same micro–Raman system, The α–AlPO4–moganite sample used in this study was and the required voltages and currents from the DC pow- from the same batch as that used in our previous crystal er supply were recorded. Then, a relation between the structure study (Kanzaki and Xue, 2012). In short, the generated temperature and required heating power is fit- sample was synthesized from dried reagent–grade AlPO4 ted with a second order polynomial equation. This equa- and was treated at 5 GPa and 1500 °C for 1 h using a tion was used to estimate the heating power necessary for multi–anvil high pressure device installed at IPM. The the desired temperature. The accuracy of the estimated recovered sample was a fine–grained (a few µm) sintered temperature would be 10–20 °C judging from reproduci- material, and our Rietveld refinement of the sample bility of the current and voltage and also repeated checks proved that the sample is mainly composed of α– of the calibration materials, but the precision of relative AlPO4–moganite with trace corundum and an unknown temperature in a single heating experiment is much better phase (Kanzaki and Xue, 2012). within a few degrees. α–AlPO4–moganite is a high pressure phase and is A small sintered fragment of α–AlPO4–moganite not a stable phase at ambient pressure (Kanzaki and Xue, was placed in the center of a hole in the heater (half–mill- 2012; Wang and Kanzaki, 2015). However, our prelimi- ed). For in–situ measurements, temperature was increased nary study revealed that AlPO4–moganite can be heated first in a step of 25 °C in a few seconds and was then kept at ambient pressure up to near 800 °C, and conversion to for 5 minutes to ensure thermal equilibrium before a Ra- berlinite (the stable quartz–like phase at ambient pres- man spectrum was acquired with a one–minute exposure sure) was observed at around 800 °C. In order to avoid time. This procedure was repeated up to 600 °C. Near complications due to the formation of berlinite, the pres- the transition temperature, additional measurements from ent experiment was limited to below 600 °C, and no ber- 370 to 430 °C with a 10 °C–step were also conducted linite wasAdvance detected. Publicationduring both heating and cooling.Article Unpolarized Raman spectra were acquired using a Relative wavenumbers in reported spectra were cali- Phase transition of AlPO4–moganite by in–situ Raman spectroscopy 3 brated against the Raman shifts of a synthetic coesite sample using a third–order polynomial equation, which were precisely calibrated against Ar+ plasma lines of a 488.123 nm Ar ion laser.
Details
-
File Typepdf
-
Upload Time-
-
Content LanguagesEnglish
-
Upload UserAnonymous/Not logged-in
-
File Pages9 Page
-
File Size-