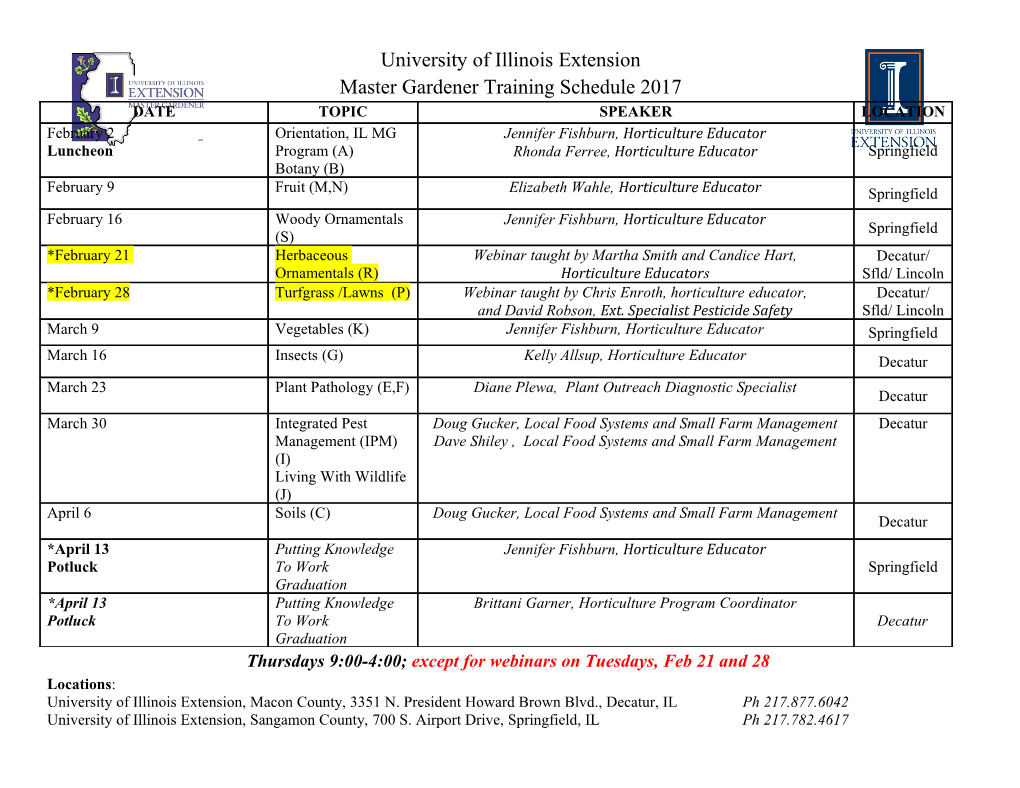
Strong relationship between elemental stoichiometry and metabolome in plants Albert Rivas-Ubacha,1, Jordi Sardansa, Miriam Pérez-Trujillob, Marc Estiartea, and Josep Peñuelasa aGlobal Ecology Unit, Centre for Ecological Research and Forestry Applications-Centre for Advanced Studies of Blanes-Spanish National Research Council, Universitat Autònoma de Barcelona, Bellaterra 08193, Barcelona, Catalonia, Spain; and bService of Nuclear Magnetic Resonance, Faculty of Sciences and Biosciences, Universitat Autònoma de Barcelona, Bellaterra 08193, Barcelona, Catalonia, Spain Edited by Christopher B. Field, Carnegie Institution of Washington, Stanford, CA, and approved January 30, 2012 (received for review October 3, 2011) Shifts in the elemental stoichiometry of organisms in response to affect the elemental content, stoichiometry, and metabolome of their ontogeny and to changing environmental conditions should be organisms (3), but most metabolomic studies have not considered related to metabolomic changes because elements operate mostly as these effects (2, 19). We hypothesized that stoichiometric and parts of molecular compounds. Here we show this relationship in metabolomic studies of plants in different ontogenetic stages or leaves of Erica multiflora throughout their seasonal development exposed to different environmental conditions should reveal an and in response to moderate experimental field conditions of organism’s flexibility in modulating its stoichiometry and metab- drought and warming. The N/P ratio in leaves decreased in the met- olome to maintain optimal fitness under different conditions. We abolically active growing seasons, coinciding with an increase in the hypothesized that seasonal differences in the metabolome should content of primary metabolites. These results support the growth- be similar to the shifts that occur in individuals growing under rate hypothesis that states that rapidly growing organisms present varying conditions of temperature and water availability. low N/P ratios because of the increase in allocation of P to RNA. The We conducted a stoichiometric and metabolomic study of the foliar N/K and P/K ratios were lower in summer and in the drought plant Erica multiflora, a Mediterranean shrub, during different treatment, in accordance with the role of K in osmotic protection, and ontogenetic periods and exposed to field conditions of moderate coincided with the increase of compounds related to the avoidance warming (0.9 C) and drought (19% reduction of soil moisture). of water stress. These results provide strong evidence of the relation- We thus tested our hypothesis that seasonal and climatic changes ECOLOGY ship between the changes in foliar C/N/P/K stoichiometry and the would force organisms to adjust both their C/N/P/K biomass changes in the leaf’s metabolome during plant growth and environ- stoichiometry and their metabolome in an interrelated way to mental stress. Thus these results represent a step in understanding maintain optimal performance under each specific condition. the relationships between stoichiometry and an organism’s lifestyle. Elemental stoichiometry should determine an organism’s capac- ity to build molecules and thus to shape metabolomic responses, climate change | metabolomics | ecometabolomics | Mediterranean climate so an organism’s metabolomic adjustments should determine the C/N/P/K biomass stoichiometry, and the seasonal and climatic he ratios of C/N/P concentrations in the environment and changes in environmental ratios of C/N/P/K availability should Tbiomass have statistically significant relationships with traits of influence an organism’s metabolomic responses. an organism’s lifestyle and even seem to influence the structure and function of ecosystems (1, 2). The growth-rate hypothesis Results (GRH), one of the central paradigms of ecological stoichiometry Seasonal Stoichiometric and Metabolomic Changes. The foliar con- (3, 4), proposes that growing organisms must increase their allo- centrations of C, N, P, and K and their respective ratios (C/N, C/P, cation of P to RNA to meet the elevated demands for the synthesis C/K, N/P, N/K, and K/P) changed with the seasons (mixed model of proteins required for growth. Low ratios of environmental N/P analyses). The lowest N/P, C/P, and C/N ratios were found in and C/P favor species with very high rates of growth (5) and may spring, whereas summer leaves showed the highest K/P and the induce shifts in species communities (6, 7). The GRH has strong lowest N/K concentration ratios (Table S1). Almost all the eluci- fi experimental support in freshwater ecosystems (2), with a few dated polar and nonpolar compounds (Fig. 1) showed signi cant P < exceptions related to the allocation of nutrients to functions other seasonal differences in concentration (mixed model analyses; than growth (8–10). In terrestrial ecosystems, however, the direct 0.05) (Table S1). Spring leaves had the highest concentrations of application of the GRH frequently fails or is accomplished in- polar metabolites, such as alanine, glutamine, asparagine, threo- α β completely (2, 11). Apart from investing N and P in growth, ter- nine, -glucose, -glucose, and sucrose. In contrast, spring leaves restrial plants can invest important amounts of these nutrients to had the lowest concentrations of lipids and secondary metabolites, other functions, such as storage, defense, and mechanisms of stress such as terpene compound 1 and derivatives of p-coumaric acid. avoidance. Therefore the phenotypic responses in these other Multivariate analysis of variance (MANOVA) analysis showed a significant interaction between stoichiometric and metabolomic basic organismal functions should be considered when assessing F P < the relationships of C/N/P ratios with an organism’s metabolome variables and seasons ( 3,13484 = 71.6, 0.0001, Table S2). Thus, and lifestyle and with the structure and function of ecosystems (2, different distributions of global metabolomic and stoichiometric fi values were observed among seasons. Permutational MANOVA 12, 13). Our goal was to consider the rst step of such relation- fi ships, (i.e., to link stoichiometry to the metabolome). (PERMANOVA) analysis also showed signi cant global differ- The metabolome is the entirety of small molecules present in an organism as the final expression of its genotype (14) and can be ’ Author contributions: A.R.-U., J.S., M.E., and J.P. designed research; A.R.-U., J.S., and considered as the organism s chemical phenotype (12). Metab- M.P.-T. performed research; A.R.-U., M.P.-T., M.E., and J.P. contributed new reagents/ olomics has been applied recently to physiological and ecological analytic tools; A.R.-U., J.S., and J.P. analyzed data; and A.R.-U., J.S., M.P.-T., and J.P. wrote studies to assess the physiological status and functions of organ- the paper. isms, including their energetic and oxidative states; functions of The authors declare no conflict of interest. growth, defense, storage, and reproduction; and mechanisms of This article is a PNAS Direct Submission. stress avoidance and health (15–18). 1To whom correspondence should be addressed. E-mail: [email protected]. Global climate change and the marked ontogenic and seasonal This article contains supporting information online at www.pnas.org/lookup/suppl/doi:10. variation throughout the year in most regions of the world should 1073/pnas.1116092109/-/DCSupplemental. www.pnas.org/cgi/doi/10.1073/pnas.1116092109 PNAS Early Edition | 1of6 Downloaded by guest on September 29, 2021 Fig. 1. Typical 1H NMR spectra of polar (water–methanol) and nonpolar (chloroform) extracts of E. multiflora leaves. Assignments of signals to metabolites are indicated in blue in the polar profile. A number has been assigned to each metabolite and to overlapped signals: 1, α-glucose (αG); 2, β-glucose (βG); 3, sucrose (Suc); 4, alanine (Ala); 5, asparagine (Asp); 6, glutamine (Gln); 7, leucine (Leu); 8, isoleucine (Ile); 9, threonine (Thr); 10, 6-deoxypyranose; 11, 4- hydroxyphenylacetate; 12, malate; 13, maleate; 14, citrate; 15, 3-amino-4-hydroxybutyrate; 16, N-acetyl group; 17, quinic acid (Q.ac); 18, tartaric acid (T.ac); 19, arbutin (Arb); 20, choline (Ch); 21, 1,2-propanediol; 22, γ-hydroxybutyrate; 23, lactate. 30–55: Overlapped signals: 30, 11+15; 31, 5+11; 32, 5+11+13; 33, 6+21; 3, 12+unknown; 35, 15+22; 36, 16+19; 37, 3+16+19; 38, 6+16; 39, 1+2+3+16+18; 40, 1+2+3+5+16+18; 41, 1+2+3+16; 42, 11+14; 43, 9+14+22; 44, 13+14; 45, 1+2+9; 46, 1+2+3+18; 47, 1+2+3+18+19; 48, 1+2+3+18+19+20; 49, 1+2+3+9+18+21; 50, 1+2+3+unknown; 51, 1+2+18; 52, 1+2+9+18; 53, 1+2+6+18; 54, 2+20; 55, 2+3+18+19. Assignments of signals to metabolites are indicated in gray in the nonpolar profile. Letter codes have been assigned to each nonpolar region or metabolites. A, C, D, and F, fatty acid spectrum regions; B, linoleyl fatty acid region; E and L, unsaturated fatty acid regions; G, free fatty acids region; H, polyunsaturated fatty acids region; I, diacylglicerid and triacylglicerid region; J, triacylglicerid 2 region; K, triacylglicerid 1 region; M, 1,2 diac- ylglicerid region; N, polyphenols region; O, aldehydes group region; Ac, acetyl group; DGA, 1,2-diacylglicerid; FAI, fatty alcohols; P1, polyphenol derived 1 of p-coumaric acid; P2, polyphenol derived 2 of p-coumaric acid; Ter, terpene compound 1; TGA1, triacylglicerid 1; TGA2, triacylglicerid 2; U1, unknown com- pound 1; U2, unknown compound 2. ences in metabolomic and stoichiometric variables among seasons The MANOVA analysis showed a significant interaction be- (pseudo-F3,68 = 29.3, P = 0.01, Table S2). tween stoichiometric and metabolomic variables and treatments The seasonal principal component analysis (PCA) with all the (F2,13484 = 5.14, P = 0.01). Thus different distributions of global stoichiometric and metabolomic data resulted in a first principal metabolomic and stoichiometry values were observed among component (PC1) separating the foliar stoichiometry and metab- climatic treatments.
Details
-
File Typepdf
-
Upload Time-
-
Content LanguagesEnglish
-
Upload UserAnonymous/Not logged-in
-
File Pages6 Page
-
File Size-