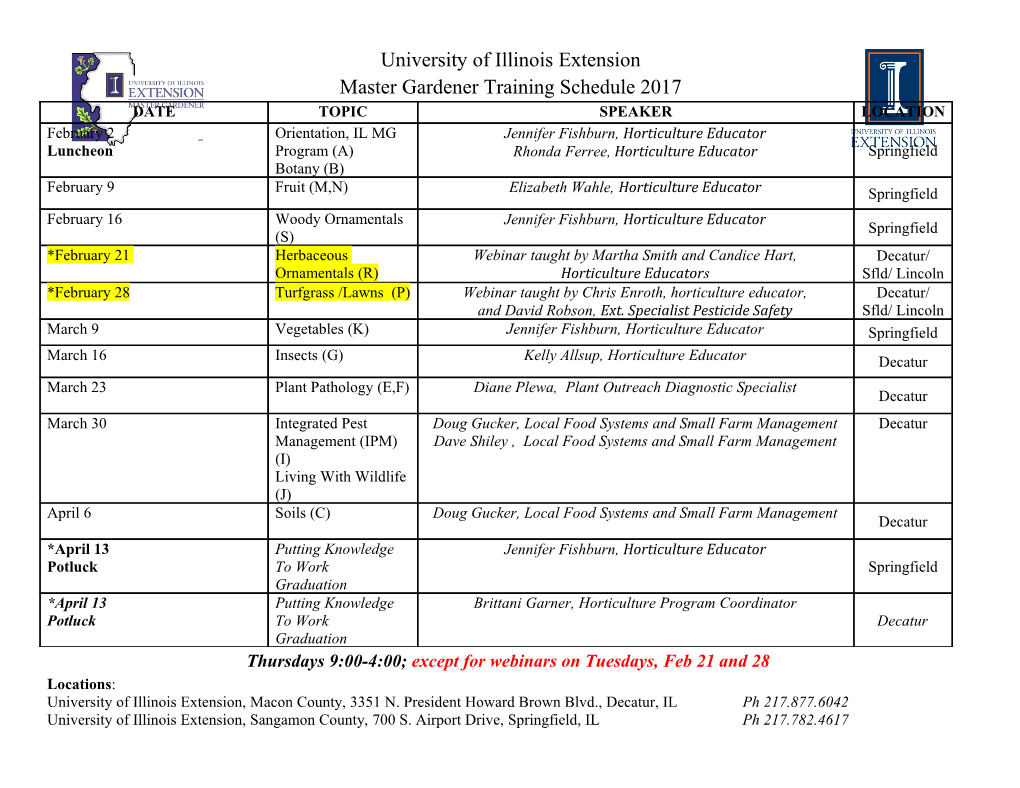
IEEE TRANSACTIONS ON ELECTRON DEVICES, VOL. 54, NO. 5, MAY 2007 907 Spintronics Michael E. Flatté, Member, IEEE (Invited Paper) Abstract—Investigations of the dynamics of spin-polarized elec- tronic current through and near materials with spin-dependent electronic structures have created a rich new field dubbed “spin- tronics.” The implications of spintronics research extend deep into the realm of fundamental material properties, yet spintronics applications have also revolutionized the magnetic-storage indus- try by providing efficient room-temperature magnetic sensors. Control of nonequilibrium spin-polarized populations of electrons through and near magnets has led to the dominance of linear (resistive) spintronic devices for magnetic readout in commercial magnetic storage. Rapid progress in understanding the funda- mental physics of nonlinear spin-polarized electronic transport in metals and semiconductors suggests new applications for spin- tronic devices in fast nonvolatile memory as well as logic devices, with or without magnetic materials or magnetic fields. Ongoing study of the interaction between such spintronic elements and optical fields, particularly in semiconductors, promises the future development of optical spintronic devices. Index Terms—Magnetoelectronics, Rashba fields, spin coher- ence, spin electronics, Spin Hall effect, spin relaxation times, spin transistor, spin transport, spintronics, spin valve. Fig. 1. (a) High-resistance and (b) low-resistance geometry of CIP GMR. The I. INTRODUCTION spacer region is a nonmagnetic metal. (c) High-resistance and (d) low-resistance geometry of CPP GMR (if the spacer region is a nonmagnetic metal) or TMR N 1988, during an investigation of the properties of multi- (if the spacer region is an insulator). Arrows indicate the magnetization I layers of magnetic and nonmagnetic metallic materials, a direction of the magnetic layers. For applications, one ferromagnetic layer is dramatic dependence of the electrical resistance on the mag- typically “pinned” through shape anisotropy and may be exchange-biased [10] and the other layer is reoriented by the external magnetic field. netization orientation of neighboring layers (parallel or anti- parallel) was reported and named “giant magnetoresistance” (GMR) [1], [2]. Within a couple of years the “spin valve” on spin-dependent electronics, which came to be called “spin- had been introduced [3], with two metallic magnetic layers tronics.” Within this field of spintronics (in its area of over- separated by a nonmagnetic spacer. Shortly thereafter, room- lap with magnetoelectronics), a fundamental physics discovery temperature magnetic-field sensors had been made [4] from came to drive a large commercial sector (2005 sales exceeding spin valves which were superior to previous state-of-the-art $3 billion) within a decade of discovery. devices based on anisotropic MR (AMR). IBM introduced The initial discovery of GMR was for a configuration (shown GMR-based magnetic media read heads into its commercial in Fig. 1(a) and (b) for a spin valve) called “current-in-plane” disk-drive products in 1997, and soon, all disk-drive companies GMR (CIP GMR). Shortly thereafter, a simpler experimental were offering GMR-based read heads instead of AMR-based geometry was investigated (Fig. 1(c) and (d), called the “current heads. Out of a field treating the effects of magnetic fields perpendicular-to-plane” (CPP) configuration [5], [6]), which on electronic transport (“magnetoelectronics,” which includes yielded a much larger value than in CIP GMR of the MR AMR and many other effects) was rapidly born a field focusing (the percent difference in resistance for parallel and antiparallel orientations of the two ferromagnetic regions in the spin valve). In each of these configurations, the two ferromagnetic regions are separated by a region of nonmagnetic metal, typically with a higher conductivity than either of the two ferromagnetic Manuscript received December 1, 2006; revised January 24, 2007. This work was supported by the Office of Naval Research. The review of this paper was layers. Motivated by earlier low-temperature measurements of arranged by Editor H. Morkoc. small magnetic-field effects on the tunneling resistance be- The author is with the Department of Physics and Astronomy, Department tween ferromagnetic layers [7], it was found that replacing of Electrical and Computer Engineering, and Optical Science and Technology Center, The University of Iowa, Iowa City, IA 52242-1479 USA. the nonmagnetic metal in the CPP-GMR geometry with an Digital Object Identifier 10.1109/TED.2007.894376 insulating tunnel barrier can yield large values of the MR at 0018-9383/$25.00 © 2007 IEEE 908 IEEE TRANSACTIONS ON ELECTRON DEVICES, VOL. 54, NO. 5, MAY 2007 room temperature [8]. This effect, referred to as “tunneling sential for calculations of spin transport and magnetooptical MR” (TMR), can exceed a factor of three at room temperature effects. For ordinary calculations of charge transport, the spin- [9]. Applications of such structures in magnetic-field sensors dependent character of these eigenstates is neglected. The rely on the reorientation of the magnetization of one of the two discovery of GMR itself, however, is evidence that, in some layers in a small magnetic field (the other layer is “pinned” circumstances, the spin-dependent character of electrical trans- through shape anisotropy and may be exchange-biased [10]) port can have a sufficient effect on ordinary charge transport and electrical detection of the change in resistance. The sensor to be easily detected. To identify the principal characteristics can be placed in a magnetic-storage read head, and the small of spin-dependent electronic structure important to spintronics, magnetic field can come from an encoded bit on magnetic the relevant materials can be grouped into several classes, based media (such as in a hard disk drive). Alternatively (such as in on whether they are magnetic or nonmagnetic and whether they magnetic random-access memory, or MRAM), the spin valve are metals or insulators (including semiconductors). itself can be used as a magnetic bit, with the two-memory states corresponding to antiparallel and parallel configurations. A. Magnetic Metals In the initial MRAM designs, a small magnetic field generated by a current pulse in an integrated wire (or pair of wires) is The common starting point for most analyses of spin trans- used to “write” the memory state, and readout is performed by port is the “two-channel” conduction approximation, originally measuring the resistance of the spin valve. developed for magnetic metals [24], [25] and, subsequently, The discovery of these spin-dependent effects in metallic applied to heterostructures of magnetic and nonmagnetic metals electrical transport has spawned a wide range of further inves- [26], [27]. This approach models the current as carried inde- tigations and has united previously distinct subfields of physics pendently by spin-up and spin-down carriers, with a separate [11]–[21] into the new field of spintronics. This includes spin- conductivity for each. The spin-up and spin-down carriers can dependent transport in semiconductors, spin-dependent tunnel- independently reach a local equilibrium but may be out of equi- ing through insulators, and spin-transport effects driven by the librium with each other. The rate of spin-flip processes, which spin–orbit interaction in semiconductors, oxides, and metals. couple the two channels, is much smaller than the ordinary The investigation of equilibrium and transient spin-dependent scattering rate characterizing the spin-conserving momentum phenomena using optical techniques, a rich field in the 1970s relaxation of nonequilibrium spin-up or spin-down distribu- [12], has blossomed again, particularly for magnetic insulators tions. Thus, even though metals often have mean free paths and nonmagnetic semiconductors. The discovery of semicon- much shorter than a nanometer, the spin-diffusion length can ductors that can become ferromagnetic upon dilute doping with range from several nanometers in strongly scattering magnetic magnetic atoms [15], [22], [23] may connect all these areas materials to several micrometers in metals lacking magnetic by providing electrical control of magnetic properties, spin- impurities or strong spin–orbit scattering. dependent transport, and strong magnetooptical effects, such as In this two-channel model, the bulk conductivity of spin-up Faraday rotation. The new areas of semiconductor spintronics and spin-down carriers is determined separately by the density have yet to have a commercial impact, although the properties of states at the Fermi energy EF for spin-up and spin-down found in these systems are unique and numerous theoretical carriers, so device proposals have been put forward. Here, some of the unifying themes of spintronics will be 2 σ↑(↓) = e N↑(↓)(EF )D↑(↓) (1) explored, including various regimes of spin transport in mag- netic and nonmagnetic metals, semiconductors and oxides, where D↑ ↓ is the diffusion constant for spin-up (spin-down) spin-dependent optical properties such as Faraday rotation, and ( ) carriers. The response of such a material to an applied electric electrical control of magnetic properties and spin lifetimes. The field is to flow a spin-polarized current, with a polarization treatment begins with a discussion in Section II of the spin- (defined below) that is determined by the conductivities dependent electronic properties of materials, including those characterizing
Details
-
File Typepdf
-
Upload Time-
-
Content LanguagesEnglish
-
Upload UserAnonymous/Not logged-in
-
File Pages14 Page
-
File Size-