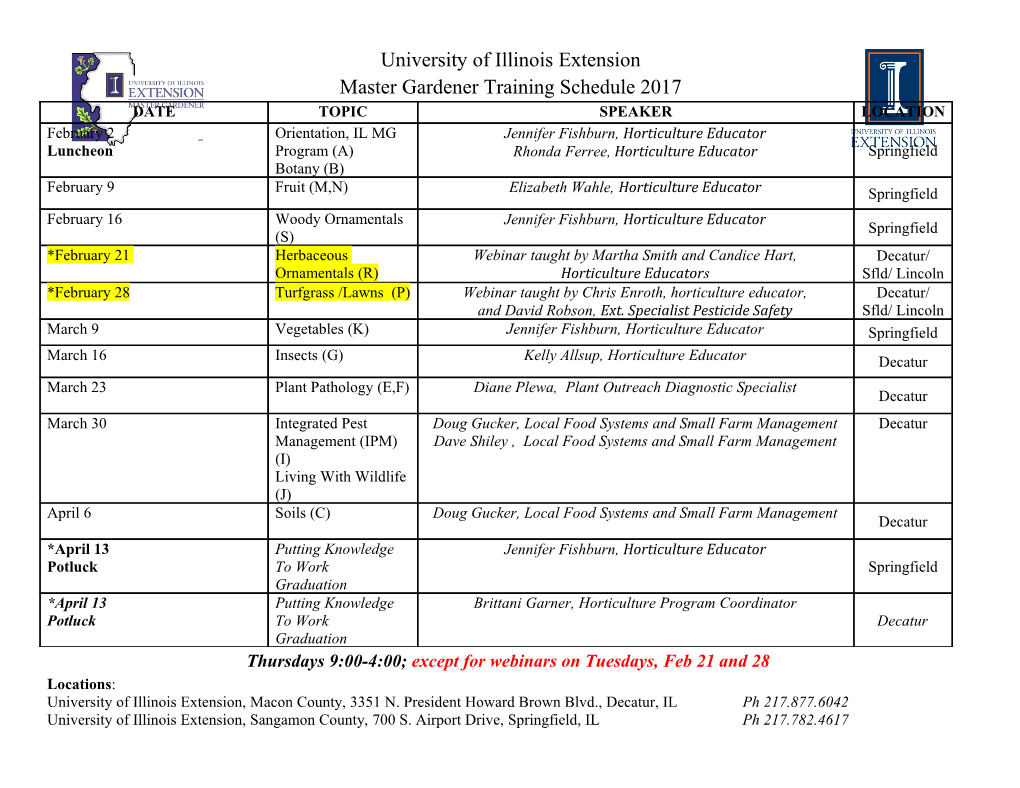
Journal of JSCE, Vol. 2, 116-135, 2014 THE DYNAMIC BEHAVIOR OF A STEEL PIPE SHEET PILE FOUNDATION IN A LIQUEFIED LAYER DURING AN EARTHQUAKE Nguyen Thanh TRUNG1, Osamu KIYOMIYA2 and Makoto YOSHIDA3 1Member of JSCE, Doctoral Student, Dept. of Civil & Env. Eng., Waseda University (Okubo 3-4-1, Shinjuku-ku, Tokyo 169-8555, Japan) E-mail: [email protected] 2Member of JSCE, Professor, Dept. of Civil & Env. Eng., Waseda University (Okubo 3-4-1, Shinjuku-ku, Tokyo 169-8555, Japan) E-mail: [email protected] 3Member of JSCE, Penta-Ocean Construction Co. Ltd. (Nasushiobara, Shikuchou 1534-1 Tochigi 329-2746, Japan) E-mail: [email protected] Various forms of damage to the bridge foundation structure in the revetment along riverbanks and sea coasts caused by liquefaction had been observed during past earthquakes. Several studies on liquefaction using physical model tests and numerical analysis have been conducted in recent years. However, few studies have investigated the seismic behavior of the foundation in a revetment with a slope. In strong earthquakes, the sloped ground is expected to be unstable, and lateral spreading of the ground may occur simultaneously with the loss of soil strength in the liquefaction layer. Moreover, in the seismic design specification (JRA-2002) of the bridge, the liquefaction verification of the foundation is stipulated for a flat ground but not for a sloped ground. Therefore, the effect of the lateral pressure of the liquefaction layer on the foundation in the revetment must be investigated further. This study aims to investigate the dynamic behavior of a steel pipe sheet pile (SPSP) foundation of a cable-stayed bridge and its effect on the per- formance of the superstructure in the revetment with a slope. A 1-G shaking table test with a scale of 1:60 was conducted on a flat model and a slope model of 15°. In addition, 2-D numerical modeling was applied in an effective stress analysis method that was used on a multi-spring model and cocktail glass model. The differences in the dynamic responses between the two models clearly illustrate the significant effect of the ground slope on the seismic behavior of the SPSP foundation and superstructure. Key Words: SPSP foundation, liquefaction, shaking table test, effective stress analysis 1. INTRODUCTION insights regarding the basic mechanisms of soil-pile interaction in liquefied soil and their effect on the Various forms of damage to the pile and caisson performance of a superstructure during liquefaction foundation structures had been observed in areas of have been understood from field observations, liquefaction in past earthquakes1), 2). Some of these shaking model tests, and numerical analysis. How- were pile failures near the bottom of the liquefied ever, most of these studies were conducted on flat layer, whereas others were pile failures near the pile ground or ground with a mild slope line for a pile head. These failures were likely caused by the liq- foundation structure. Ramin et al.6) conducted a large uefaction that occurred due to a decrease in the soil shaking table test on the pile foundation near a grav- strength and lateral movement of the liquefied layer. ity-type quay with flat ground. Haeri et al.7) and Moreover, significant damage was observed at both Ramin et al.8) investigated the response of a group of the pile body and pile head in the sites located near or piles to liquefaction-induced lateral spreading by on the revetment with a sloped surface ground along large-scale shake testing using a sloped ground with riverbanks or sea coasts3), 4), 5). This damage was an angle of 5°. In addition, Tokida et al.9) conducted likely caused by the unstable ground during lique- tests on various sloped ground models at 5° angle and faction. In recent years, many important lessons and varying slope lengths. Miyajima et al.10) performed a 116 Tower of Cable Stayed Bridge Steel Pipe Sheet Pile Foundation 11000 (SPSP Foundation) unit: mm 52000 SPSP foundation A A 95500 36456 5500 (A-A) D1500xt25 43500 12670 n=165 piles 29469 12060 15000 36456 Fig.1 Tower of Cable-stayed Bridge. shaking table test and determined that the pile re- the liquefied ground and bridge foundation during sponse depends on the sloping surface of the ground, vibration. The eigen value analysis technique was ranging from 2° to 6°. Tokimatsu et al.11), 12) studied used to investigate the dynamic characteristics of the seismic behavior of soil-pile-superstructure sys- models. Furthermore, the ESA technique was used to tem during soil liquefaction and liquefaction-induced consider the liquefaction of loose sand for both ground displacement by shaking table test. There- drained condition and undrained condition. fore, the researches simulated the dynamic behavior of pile foundation on the flat ground or with mild slope from 2° to 6°. However, the SPSP foundation, 2. SHAKING TABLE TEST a type of caisson foundation, works not only as a support structure but also as a retaining wall in the This study was conducted using the 1-G shaking revetment, that may not have been discussed before. table facility of the Penta-Ocean Construction Cor- Consequently, in this study, the behavior of SPSP poration in Japan. The shaking table test was de- foundation with a slope of 15° will be investigated. signed for both the sloped and flat models to inves- Moreover, in the current bridge seismic design tigate the difference in the dynamic response be- specification by JRA et al.13), the verification of the tween the models under liquefaction conditions, as liquefaction of the foundation structure is stipulated described in the following sections. for flat ground. The verification of liquefac- tion-induced lateral spreading is conducted for a (1) Prototype foundation that is less than 100 m from the water- The tower and superstructure of a cable-stayed front. The foundation in the revetment with a slope, bridge supported by the SPSP foundation on the whether affected by liquefaction-induced lateral ground was modeled in the shaking table test. The spreading or not, is not clearly mentioned, thus fur- outline of the tower is shown in Fig.1. The founda- ther investigations and studies are required. tion has 165 steel pipe piles with dimensions of In this study, a 1-G shaking table test with a 36.456 m length and 29.469 m width. Each steel pipe 1:60-scale model was designed for two test models of pile has a diameter of 150 cm and a thickness of 2.5 SPSP foundation to determine the behavior of the cm. bridge foundation during vibration. The first model To simplify the structure for constructing the was on a flat ground surface (denoted by the flat physical model, the superstructure-tower system of model), and the second model was on a 15°-sloped the prototype was modeled as a single degree of ground (denoted by the slope model). Additionally, a freedom system. The mass of the system includes the 2-D numerical finite element method using the ef- mass of the superstructure and tower at the top of the fective stress analysis (ESA) and eigen value analysis column. The natural frequency of the system (fL) was techniques was conducted to simulate the behavior of calculated as suggested by Yoneda14) as follows: 117 Photo 1 1-G shaking table test on the flat model. Table 1 Scaling factors of shaking model test. 100 %) ( 90 Yamagata sand λ = prototype 80 (No. 6) Parameter Scale /model 70 Length λ 60 60 Density 1 1 50 Time λ0.75 21.56 40 Stress λ 60 30 Pore water pressure λ 60 20 Displacement λ1.5 464.76 bypercentweight Pass 10 Acceleration 1 1 0 Strain λ0.5 7.75 0.001 0.01 0.1 1 10 Water permeability Grain size (mm) λ0.75 21.56 coefficient Bending stiffness λ4.5 100,387,728 Fig. 2 Grain size distribution of Yamagata sand. i height and a cross section in a tubular shape of 2.27 3EI /(h)3 cm diameter and 0.19 cm thickness. The foundation 1 f 0 (1) is a caisson made of acrylic materials with a dimen- L 2 m e sion of 49 cm width, 60.8 cm length, and 83.4 cm height. The cap at the top of the foundation is an where fL is natural frequency; me is the mass of the acrylic plate that is 60.8 cm long, 49 cm wide, and superstructure; h is the height from the bottom of the 9.8 cm thick. The footing of the pier is constructed of pier to the position of the lowest cable; α is the factor steel with dimensions of 26.6 cm length, 46.6 cm that depends on the ratio of stiffness between the width, and 18.5 cm thickness. tower and girder; and I is the area moment of inertia The ground in the models consists of a 48.8 cm of the tower. liquefiable sand layer with a relative density of 50% using Yamagata sand No. 6 (D50 = 0.3 mm) overlying (2) Test model and material properties a 74.3 cm non-liquefiable layer with a relative den- All material properties of the physical model and sity of 90%. The soil layers of the model ground were ground were scaled using a similitude law suggested constructed using the sand drop method. The sand by Iai15). Table 1 summarizes the scaling factors was gradually dropped into the vessel up to the water applied in this shaking model test. level step. However, the relative density of the Photo 1 shows a set-up of the flat model on the non-liquefiable layer was controlled by the amount shaking table.
Details
-
File Typepdf
-
Upload Time-
-
Content LanguagesEnglish
-
Upload UserAnonymous/Not logged-in
-
File Pages20 Page
-
File Size-