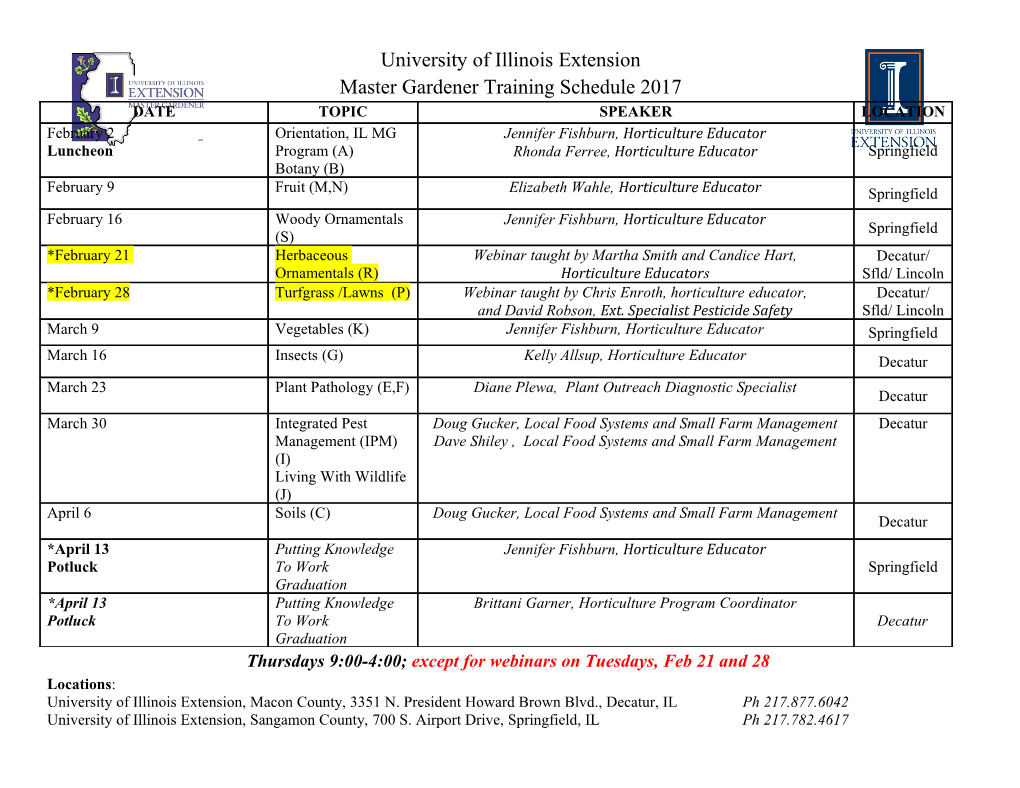
Slot Suction of the Turbulent Boundary Layer An Experimental Study Tim van der Hoeven March 28, 2013 Faculty of Aerospace Engineering ⋅ Delft University of Technology Slot Suction of the Turbulent Boundary Layer An Experimental Study Master of Science Thesis For obtaining the degree of Master of Science in Aerospace Engineering at Delft University of Technology Tim van der Hoeven March 28, 2013 Faculty of Aerospace Engineering ⋅ Delft University of Technology Copyright © Tim van der Hoeven All rights reserved. Delft University Of Technology Department Of Aerodynamics The undersigned hereby certify that they have read and recommend to the Faculty of Aerospace Engineering for acceptance a thesis entitled \Slot Suction of the Turbulent Boundary Layer" by Tim van der Hoeven in partial fulfillment of the requirements for the degree of Master of Science. Dated: March 28, 2013 Head of department: Prof. Dr. Ir. F. Scarano Supervisor: Dr. Ir. L.L.M. Veldhuis Reader: Dr. Ir. B.W. van Oudheusden Reader: Ir. E. Terry Reader: Dr. Ir. D. Ragni Abstract This study examines the effects of slot suction on the mean characteristics of a two-dimensional turbulent boundary layer. Previous studies on this subject have mainly focused on low Reynolds number boundary layers (i.e. Reθ ≤ 2,000). Furthermore, numerical tools are often not able to accurately predict the effects of slot suction. To grasp the effects of slot suction at conditions more relevant to engineering problems, the present research focuses on a turbulent boundary layer with a Reynolds number of Reθ ≈ 10,000. An experimental investigation has been conducted in the W-tunnel facility at the Delft Univer- sity of Technology. Two-component planar particle image velocimetry measurements have been performed for boundary layer slot suction at a zero pressure gradient and at an adverse pressure dp gradient of dx ≈ 200 [Pa/m]. For these cases, the Reynolds number based on the momentum thick- ness, for the undisturbed boundary layer was 10,100 and 11,600 respectively. Furthermore, for the adverse pressure gradient case, comparative measurements have been conducted for distributed suction through a porous plastic and a perforated plate. The results from these measurements are presented in terms of the mean velocity field, the mean pressure distribution and the boundary layer integral parameters. The result of suction on the zero pressure gradient boundary layer show good qualitative correspondence with the low Reynolds number literature. A nearly linear relation is found between the reduction in the Reynolds number based on the momentum thickness and the suction coefficient. For the shape factor this relation is found to be non-linear. The results for slot suction in the presence of an adverse pressure gradient, generally show strong similarity with the zero pressure gradient case. The change in the pressure distribution and the Reynolds number based on the momentum thickness, relative to the situation without suction is similar as for the zero pressure gradient case. The reduction in shape factor is also found to be non-linear. However, the reduction is higher than for the zero pressure gradient case. The results for distributed suction through a porous plastic or a perforated plate show similar results. Compared to slot suction, the effects near the region of suction are much more gradual. This is reflected by the local differences between distributed suction and local suction, for the pressure distribution and the integral parameters. However, downstream, where the local influ- ences have vanished, the boundary layer development in terms of the integral parameters is equal for localised and distributed suction. Concluding, the results in this report form a new source of information, which can be used in the application of boundary layer suction for engineering purposes. The obtained dataset also forms a v vi Abstract valuable addition to the scarce data on suction of the turbulent boundary layer and can therefore be used for validation of numerical simulations. Acknowledgements This thesis forms the final effort to obtain a master's degree in aerospace engineering at the Delft University of Technology. I would like to thank the people who have supported me during my studies and in particular during this thesis research. First of all I would like to thank my direct supervisors at the faculty, Dr. Ir. L.L.M. Veldhuis and Dr. Ir. B.W. van Oudheusden, for their feedback and guidance during the thesis research. Furthermore I would like to thank Dr. Ir. D. Ragni, for his support during the experimental campaigns and during the evaluation of the results, without which I would not have been able to accomplish this work. I would also like to thank my colleages at Actiflow for their support and for giving me the opportunity to perform my thesis research in a fine working environment. Last, but most certainly not least, I would like to express my gratitude to my parents, friends and especially my girlfriend, Steffie, for their continuous support during my studies. Tim van der Hoeven vii Contents Abstract v Acknowledgements vii List of Figures xvii List of Tables xx Nomenclature xxi 1 Introduction 1 1.1 Background and Motivation . .1 1.1.1 Boundary Layer Suction on Wind Turbine Blades . .2 1.1.2 Findings from Literature Review . .3 1.2 Research Goals . .4 1.3 Significance . .4 1.4 Outline of the Thesis . .5 2 Theory and Background 7 2.1 Theory of a Turbulent Boundary Layer . .7 2.1.1 Boundary Layer Characteristics . .7 2.1.2 Turbulent Boundary Layer Equations . .9 2.1.3 Mean Velocity Profile . 11 2.1.4 Estimating the Friction Velocity . 13 2.2 Boundary Layer Suction . 14 2.2.1 Principle of Boundary Layer Suction . 14 2.2.2 Slot Suction in Literature . 17 2.3 Particle Image Velocimetry . 19 2.3.1 Interrogation Window Size . 19 2.3.2 Sub-Pixel Precision and Peak Locking . 20 2.3.3 Other Sources of Error . 21 2.4 Boundary Layer Suction on Wind Turbine Airfoil . 22 ix x Contents 3 Experimental Setup 23 3.1 General Setup . 23 3.2 General Measurement Procedure . 25 3.2.1 Measurement Campaigns . 25 3.2.2 Measurement Procedure . 26 3.3 Suction System . 27 3.3.1 Slot Shape . 27 3.3.2 Other Suction Media . 28 3.3.3 Suction Chamber . 29 3.3.4 Volume Measurement . 33 3.4 Particle Image Velocimetry Measurements . 34 3.5 Pressure Measurements . 36 3.6 Hotwire Measurements . 37 4 Data Processing 39 4.1 PIV Post-processing . 39 4.2 Determination of Integral Parameters . 41 4.2.1 Determination of Freestream Velocity . 42 4.2.2 Near-Wall Data Points . 42 4.3 Smoothing of the Results . 44 4.4 Image Stitching . 45 4.5 Obtaining Pressure from PIV . 46 5 Results 47 5.1 Results from Preparatory Experiments . 47 5.1.1 Two-Dimensionality of the Main Flow . 47 5.1.2 Two-Dimensionality of the Suction Velocity . 51 5.1.3 Determination of the Sample Size . 51 5.2 Slot Suction with Zero Pressure Gradient . 53 5.2.1 Measurement without Suction . 53 5.2.2 Flow Pattern due to Suction . 62 5.2.3 Effect of Suction on the Pressure Distribution . 64 5.2.4 Effect of Suction on the Integral Parameters . 68 5.3 Slot Suction with an Adverse Pressure Gradient . 72 5.3.1 Measurement without Suction . 72 5.3.2 Effect of Suction on the Pressure Distribution . 76 5.3.3 Effect of Suction on the Integral Parameters . 80 5.4 Slot Suction Compared with Distributed Suction . 83 5.4.1 Measurement without Suction . 83 5.4.2 Flow Pattern due to Suction . 85 5.4.3 Effect of Suction on the Pressure Distribution . 87 5.4.4 Effect of Suction on the Integral Parameters . 89 5.5 Influence of Slot Width . 91 5.6 Uncertainty Analysis . 93 5.6.1 Uncertainties in PIV . 93 5.6.2 Estimation of the Uncertainty in the Integral Parameters . 96 Contents xi 6 Conclusions and Recommendations 101 6.1 Conclusions . 101 6.2 Recommendations . 104 References 105 A Test Matrices 109 B Suction Rate Measurement Method 113 B.1 Pipe Flow Velocity Profile . 113 B.2 Test Setup . 115 B.3 Velocity Profile . 116 B.4 Maximum Measured Velocity . 118 C Derivation of Two-Dimensional Poisson Equation for the Mean Pressure 121 D Original Data for Slot Suction with Zero Pressure Gradient 123 E Validation of Volume Flow 125 xii Contents List of Figures 1.1 Concept of boundary layer suction at the root of the wind turbine blades [3]. .2 2.1 Displacement thickness and momentum thickness graphically displayed. Taken from [45].................................................8 2.2 Relation between shape factor and the boundary layer velocity profile. Adapted from [42]. .9 2.3 Inner, outer and overlap layer for a typical turbulent boundary layer velocity profile. 11 2.4 Schematic demonstration of Clauser plot. Note that the x-axis has a logarithmic scale. 14 2.5 The interrelation between different flow control goals. Image taken from [13]. 15 2.6 Schematic representation of effect of suction on the shape of the boundary layer. 16 2.7 Variations of the mean pressure at the wall due to slot suction observed by Park et al. [29]. The figure has been adapted to show the result for suction only. ∎: σ = 0.09, ●: σ = 0.225, ▲: σ = 0.45. Chain-dotted lines show the location of the slot. 18 2.8 Variations of the Reynolds number based on the momentum thickness due to slot suction observed by Park et al. [29]. The figure has been adapted to show the result for suction only. ∎: σ = 0.09, ●: σ = 0.225, ▲: σ = 0.45.
Details
-
File Typepdf
-
Upload Time-
-
Content LanguagesEnglish
-
Upload UserAnonymous/Not logged-in
-
File Pages152 Page
-
File Size-