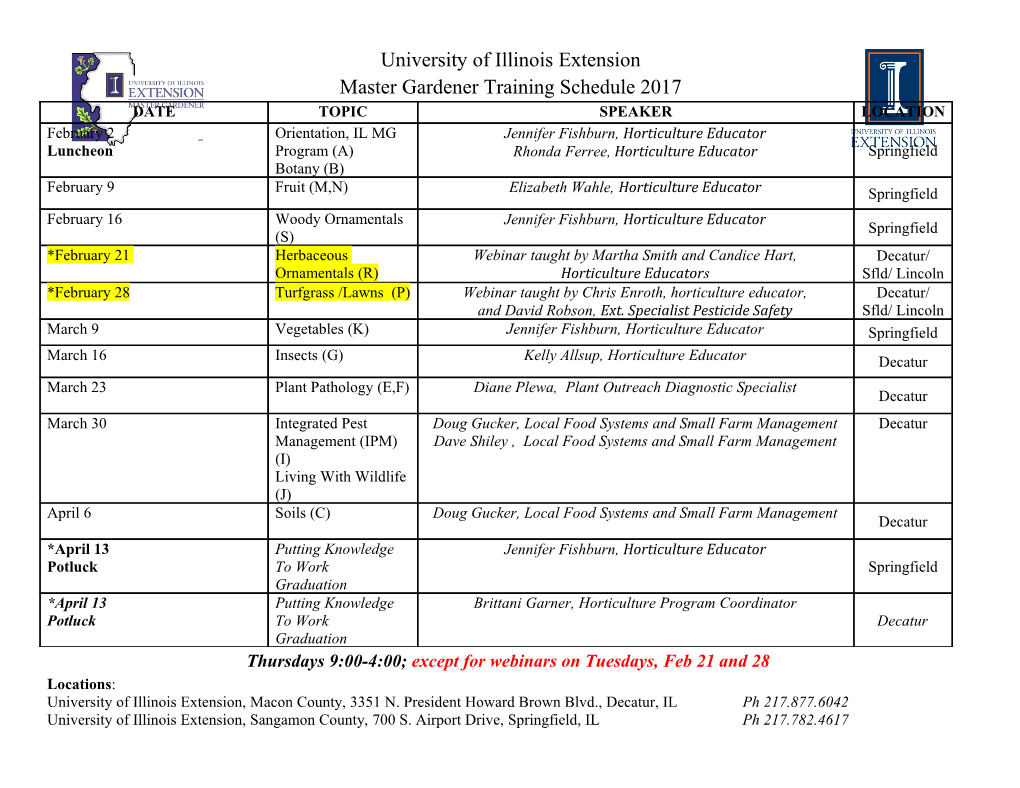
Astronomical Science Globular Clusters and the Milky Way Connected by Chemistry Bruno Dias1 stars as tracers of the history of the Milky taminated by non-cluster members and Ivo Saviane1 Way. They found that older metal-poor does not provide information on, for Beatriz Barbuy2 stars have more elliptical orbits while example, any star-to-star chemical abun- Enrico V. Held³ younger metal-rich stars move in circular dance variations. Nowadays it is well Gary Da Costa4 paths. They concluded that the proto- known that stars in most globular clus- Sergio Ortolani5,3 Milky Way had collapsed by the time of ters are split into at least two generations, Marco Gullieuszik3 the formation of the first stars and a few evident from variations in the abundances hundred million years later the collapsed of light-elements such as Na, O, Mg, Al, gas achieved circular motion and formed C, N. Further, we now know that bright 1 ESO new stars. This was the beginning of horizontal branch stars can be bluer or 2 Instituto de Astronomia, Geofísica e the field now called galactic archaeology. redder depending on their He abundance. Ciências Atmosféricas, Universidade de The current challenge is to find a model São Paulo, Brazil Another important step was provided by that connects the variations in Na, O, Mg, 3 INAF, Osservatorio Astronomico di Searle & Zinn (1978), who found no trace Al, C and N with the variation in He. Age Padova, Italy of a radial metallicity gradient for the halo and total metallicity also play a role in 4 Research School of Astronomy & Astro- globular clusters, and suggested that halo determining horizontal branch morphol- physics, Australian National University, globular clusters formed in proto galactic ogy (see reviews by Gratton et al., 2004, Australia fragments that were accreted to build the 2012). Therefore, in order to understand 5 Dipartimento di Fisica e Astronomia, Milky Way halo, after the collapse of the the formation of stars in globular clusters, Università di Padova, Italy Bulge. Since then many works have been it is clearly important to derive chemical devoted to the use of globular clusters abundances of individual cluster stars. in the study of galactic archae ology. In There are two ways to study galaxy for­ particular, with the advent of tele scopes However, only about 60% of Milky Way mation and evolution: one is to observe in space, and larger ground-based tele- globular clusters (of which 157 are cur- a large number of galaxies at a variety scopes, such studies are able to spatially rently known [Harris, 1996]) have had their of redshifts, the other is to observe in resolve stars in these clusters and also metallicities derived on a homogeneous detail just a few nearby galaxies. The to obtain spectra of individual stars, even scale based on consistently obtained precision achievable by the latter method for the most distant clusters. Saviane et integrated or resolved spectroscopy. But enables the galactic history, including al. (2012) present a summary of this work. only ~ 50% of these deter minations are the formation and early evolution, to be Figure 1 illustrates the current capacity based on spectroscopy of individual studied. Globular clusters provide tar­ to observe individual stars in clusters in a gets for the second method. We show broad context. how the chemical content of Milky Way Figure 1. (a) All-sky view centred on the Milky Way globular clusters can be used to place Plane in an Aitoff projection from Mellinger (2009) them on a timeline charting the history Digging into the clusters with a Galactic coordinate grid. The globular clusters of our Galaxy. The results suggest that studied here are identified by letters according to their populations, namely (B)ulge, (D)isc, (I)nner halo, different α­elements trace different pro­ Almost 40 years after the work of Searle and (O)uter halo. (b) Zoomed-in image of the globular cesses of Milky Way chemical evolution. & Zinn, it is legitimate to ask what there cluster 47 Tucanae (near-infrared image taken with is left to study. In many investigations VISTA: ESO image eso1302a), which can be seen that followed Searle & Zinn, astronomers with the naked eye in a dark sky. (c) Detail from the same image as (b) of an outer region of 47 Tucanae, The seminal work of Eggen, Lynden-Bell observed the integrated light of all the showing its brightest bluer and redder stars; the latter & Sandage (1962) was the first to use globular cluster stars, which can be con- stars were analysed in this work. The Messenger 165 – September 2016 19 Astronomical Science Dias B. et al., Globular clusters and the Milky Way Connected by Chemistry stars (Carretta et al., 2009). Furthermore, :%D'< l l :%D'< l :%D'< :%D'< l :%D'< high-resolution spectroscopic metallici- ' " " ' # ties are available for only ~ 25 % of them @ A (Pritzl et al., 2005). Not only is this num- ber of clusters low but the sample is biased, given that it includes only a few metal-rich Bulge clusters. We selected 51 globular clusters that M HN represent well the Milky Way Bulge, Disc, @S and Halo populations, and which cover BNLOHK the full range of metallicity, total mass, dis- tance and reddening values, in order to ' increase the statistics and generate an unbiased sample. In particular, the cluster :%D'< red giants in half of our sample are very faint owing to substantial foreground dust or large distances, and are therefore poorly studied (Dias et al., 2016). The pre- sent work increases to ~ 70% the fraction of clusters with known spectroscopic m CDW Q m CDW Q metallicity derived on the same scale. High-resolution spectroscopic observa- tions would be too time-consuming if devoted to the sample of about 800 red giant stars in 51 globular clusters that we have studied, especially for the fainter 1DRHCT@KR targets. We instead used a lower resolu- tion (R ~ 2000) spectrograph, which con- sequently allows higher signal-to-noise ratios to be achieved with lower integra- :%D'<"BNLOHK@SHNM :%D'<#BNLOHK@SHNM tion times. This approach also enables us to observe more targets and have a more for the globular clusters in common: Figure 2. (a) Comparison of metallicities of 132 clus- complete sample, including fainter tar- the root mean square of the differences ters common to the metallicity scales of Carretta et al. (2009) and Harris (1996), C09 and H10 respectively. gets. For the analysis we were able to is within 0.08dex and presents no trend The best fit and σ1 limits are shown by red continuous apply full-spectrum fitting that is intrinsi- with abundance. Therefore there is no and dotted lines, respectively. (b) The same as (a) but cally reddening-free (Dias et al., 2015). need to re-calibrate our FORS2 results for 151 clusters common to our abundance scale The method has the advantage that it can (Dias et al., 2016). (Dias et al., 2016a) and that of Harris (1996). Details of the fits are given in each panel. be applied to red giants in extragalactic globular clusters in the future. Carretta et al. (2009) applied their metal- licity scale, based on UVES data, to all our compilation of abundances and those previous sets of cluster metallicity and from Harris, with a mild linear trend that FORS2 data averaged them. Their scale is limited in should be applied to the Harris (1996) the metal-rich tail because they did not values. The comparison with Carretta et The FOcal Reducer / low dispersion Spec- observe clusters in this regime. Harris al. confirms the discrepancy for the trograph (FORS2) in its medium-resolution (1996) produced a compilation of cluster metal-rich clusters as expected, and and multi-object mode (Appenzeller et al., metallicities based on spectroscopy and reveals a few outliers. We have increased 1998) is consequently more suitable for photometry, and made an effort to put the fraction of clusters with known (spec- our purposes than the Fibre Large Array them all on a homogeneous scale, simi- troscopic and photometric) metallicities Multi Element Spectrograph (FLAMES) larly to Carretta et al. We followed the from 84 % (Carretta et al.) to 97% of the facility (Pasquini et al., 2002) combined same steps, with the advantage that we total Milky Way globular cluster sample, with the Ultra-violet Visible Echelle Spec- have 51 clusters in our sample (compared improving coverage of the metal-rich tail. trograph (UVES). The first question that to 19 for Carretta et al.) as references arises is: are we losing precision in the for scaling previous work. Importantly, determination of chemical abundances our clusters cover the full metallicity Field versus cluster stars with this choice? We were able to derive range: the catalogue of globular cluster metallicities from FORS2 spectra in very abundances can be downloaded1. Fig- It has been known for quite some time good agreement with UVES metallicities ure 2 shows good agreement between that the ratio of α-elements over iron [α/Fe] 20 The Messenger 165 – September 2016 is larger at the beginning of a galaxy’s life, as a result of early chemical enrichment by core-collapse supernovae. Some time later, estimated to be around 0.5 to 2 Gyr, thermonuclear type Ia supernovae pro- duce large amounts of iron, which causes [ /Fe] to decrease. The time when the α < second event starts to be important is a %D key piece of information for understand- F ing the efficiency of star formation in a :, galaxy. In the case of the Milky Way, the chemistry of field stars indicates a time- scale of about a billion years as indicated by the depletion in [α/Fe] (e.g., Matteucci @ & Recchi, 2001).
Details
-
File Typepdf
-
Upload Time-
-
Content LanguagesEnglish
-
Upload UserAnonymous/Not logged-in
-
File Pages3 Page
-
File Size-