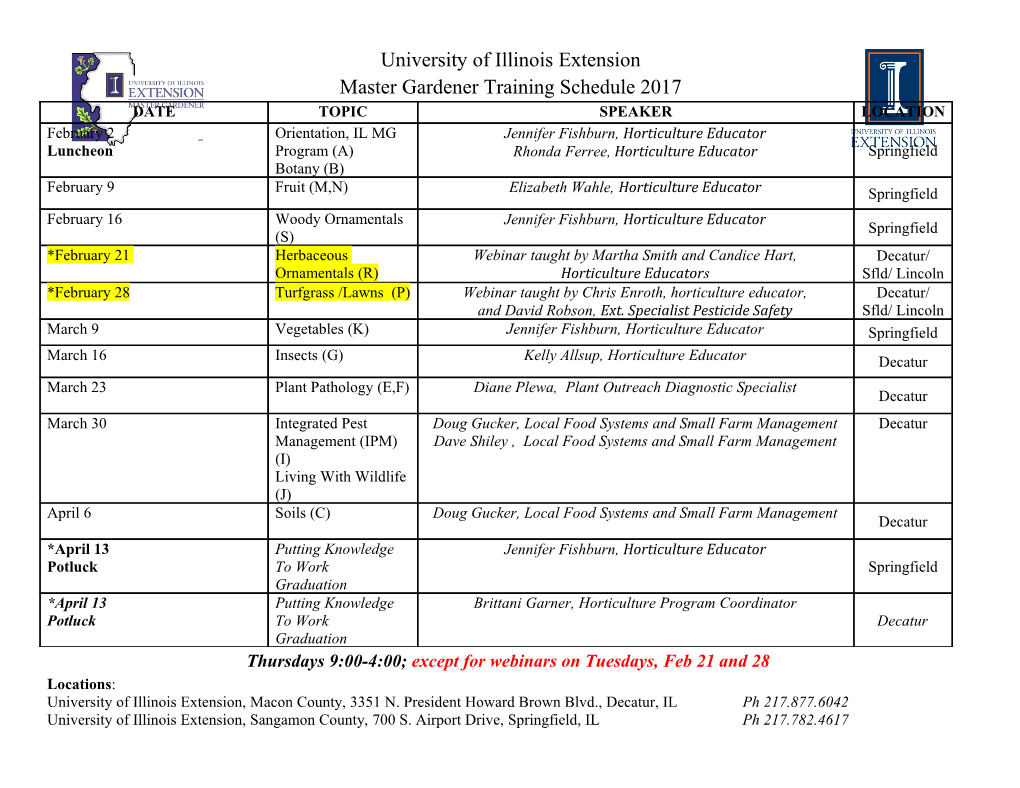
The influence of the Hel OCEANOLOGIA, 47 (4), 2005. pp. 567–590. upwelling (Baltic Sea) on C 2005, by Institute of nutrient concentrations Oceanology PAS. and primary production KEYWORDS –theresultsofan Baltic Sea ecohydrodynamic model* Upwelling Ecohydrodynamic modelling Nutrients Primary production Marek Kowalewski Institute of Oceanography, University of Gdańsk, al. Marszałka Piłsudskiego 46, PL–81–378 Gdynia, Poland; e-mail: [email protected] Received 21 February 2005, revised 10 October 2005, accepted 20 October 2005. Abstract An ecohydrodynamic model was used to investigate the effect of the Hel upwelling on nutrient concentrations, primary production and phytoplankton biomass. The model covered the whole Baltic Sea with a 5 NM grid spacing and the Gulf of Gdańskwith a 1 NM grid spacing. Validation indicated good agreement between model results and measurements in the GdańskDeep, and slightly weakerconcord for the Hel upwelling area. The vertical nutrient fluxes associated with up- and downwelling in the Hel region were simulated for two 30-day periods in 2000. The nutrient input resulting from long-term upwelling is comparable to the load carried into the Gulf of Gdańskby the Vistula (Wisła), the largest river in the vicinity. Performed at times when upwelling was almost permanent, the simulations showed elevated nutrient concentrations in surface waters. This was especially distinct in spring when primary production and phytoplankton biomass were both higher. In late summer, however, upwelling caused primary production to decrease, despite the elevated nutrient levels. * This research was supported by the State Committee for Scientific Research, Poland (grant No 6 P04G 061 17). Editing assistance of the article was provided by BALTDER (EVK3-CT-2002-80005), funded by the European Commission under the 5th Framework Programme. The complete text of the paper is available at http://www.iopan.gda.pl/oceanologia/ 568 M. Kowalewski 1. Introduction Previous papers on coastal upwelling in the Baltic Sea have usually focused on descriptions of its occurrence and the conditions of its gener- ation. Satellite observations provide detailed information on such events (Horstman 1983, 1987, Gidhagen 1984, 1987, Bychkova & Viktorov 1987, Bychkova et al. 1988, Urbański 1995, Krężel 1997a, Siegel et al. 1999). In recent years some attempts at modelling upwelling have also been undertaken. Lass et al. (1994) and Fennel & Seifert (1995) studied the dynamics of the upwelling that tookplace northwest of R¨ugen. A three- dimensional hydrodynamic model (Kowalewski 1998) was used to examine the areas where upwelling occurs, the meteorological preconditions for such events in the Baltic Sea and their frequency (Kowalewski & Ostrowski 2005, this volume). Upwelling along the Polish coast has been simulated by Jankowski (2002). Myrberg & Andreyev (2003) determined an upwelling index for different parts of the Baltic, based on a ten-year model simulation. Surface water nutrients are usually consumed or even depleted as a result of the life functions of phytoplankton. Upwelling could make that deficit good, since it raises nutrients from deeper waters. One could put forward the hypothesis that since upwelling fertilises the surface water, it should contribute to an increase in primary production and consequently, in the biomass of phytoplankton and zooplankton. Studies of the chemical and biological effects of upwelling in the Baltic Sea began not long ago, but they have often yielded contradictory results. Nˆommann et al. (1991) reported an increase in primary production near Hiiumaa Island within some days of an upwelling event. The raising of nutrients by upwelling along the Hanko Peninsula, lying between the Gulf of Finland and the Baltic Proper, was described by Haapala (1994). Uitto et al. (1997) noted that this process was accompanied by a decrease in primary production. Siegel et al. (1999) found from satellite observations and ecological modelling that after the first spring phytoplankton bloom, when surface nutrient salts were depleted, coastal upwelling sufficed to support the bloom. However, satellite images indicated a lower chlorophyll a concentration during spring upwelling events (Semovski et al. 1999, Krężel et al. 2005, this volume). Cold waters raised to the surface prevented cyanobacteria blooms in summer (Siegel et al. 1999) and increased the chlorophyll a concentration in the Hel upwelling area in early autumn (Krężel et al. 2005, this volume). Ennet et al. (2000) used an ecohydrodynamic model to study the influence of upwelling on phytoplankton blooms in the Gulf of Riga. Kostrichkina & Yurkovskis (1986) noted an increase in zooplankton biomass in the open sea 2–3 weeks after an upwelling occurrence, off the eastern shores of the Baltic Proper. The influence of the Hel upwelling (Baltic Sea) on nutrient . 569 The present workinvestigated the vertical transport of nutrients as a result of upwelling along the Hel Peninsula (Gulf of Gdańsk). The ProDeMo ecohydrodynamic model (Jędrasik1997, Ołdakowski& Renk 1997, Ołdakowski et al. 2005, this volume) describes the dynamics of organic matter production and decomposition in terms of nutrient cycles in the sea. It was applied here to determine nutrient fluxes and to study the effect of the Hel upwelling on primary production and phytoplankton biomass. 2. The ProDeMo model Any study of coastal upwelling in the Baltic Sea is fraught with difficul- ties owing to the small extent and irregular occurrence of such events. They take place when winds blow from certain directions; the Hel upwelling, for example, makes its appearance when winds are from the east, south-east and south. The poor reliability of long-term wind forecasts is a further ob- stacle to the efficient planning of research cruises. Moreover, since upwelling occurs in a narrow coastal zone, it is rarely reported during routine monito- ing. It is therefore satellite detection methods, and recently also numerical modelling, that are mostly used nowadays for investigating upwelling. Developed at the Institute of Oceanography, University of Gdańsk, the ProDeMo ecohydrodynamic model was used in the present work (Ołdakowski & Renk 1997, Ołdakowski et al. 2005, this volume). A three- dimensional numerical model describing biogeochemical processes in the sea, it is integrated with a hydrodynamic model (Kowalewski 1997) and allows the horizontal and vertical transport due to advection and diffusion to be calculated. The latter model had been used in earlier studies of upwelling dynamics in the Baltic, and the validated model results showed good agreement with satellite observations (Kowalewski 1998, Kowalewski & Ostrowski 2005, this volume). The two models cover the Baltic Sea and the Gulf of Gdańskwith respective numerical grids of c. 5 and 1 NM (Fig. 1). A numerical grid with a sigma-transformation enabled the vertical profile at any point in the sea, irrespective of depth, to be divided into the same number of layers – in this case, 18 layers of different thickness. Thinner layers were adopted to obtain a better representation of the surface and bottom boundary layers. The ProDeMo model addresses 15 state variables that can be classified into a number of functional groups: nutrients, phytoplankton, zooplankton, organic detritus and dissolved oxygen. Nutrients include nitrate nitrogen (N-NO3), ammonium nitrogen (N-NH4), phosphate phosphorus (P-PO4) and silicate silicon (Si-SiO4). The nitrogen, phosphorus and silicon cycles are much the same with respect to exchange with the bottom sediments and the air. The nitrogen cycle involves mineralisation, nitrification, 570 M. Kowalewski Hel upwelling area P1 Baltiysk Straits 54o40' Gulf of Gdañsk Hel latitude N Vistula Spit Gdañsk o Vistula Lagoon 54 20' Vistula 18oo30' 19 00' longitude E Baltic Fig. 1. The modelled areas – the Baltic Sea and the Gulf of Gdańsk denitrification and uptake by phytoplankton, the changes in phosphorus concentration are described by mineralisation, uptake by phytoplankton, and absorption-desorption on suspension, and the silicon cycle includes mineralisation and uptake by phytoplankton (Ołdakowski et al. 2005, this volume). The phytoplankton was divided into two groups – diatoms and other forms. The biomass changes in these groups were due to phytoplankton growth (in relation to temperature and light intensity), respiration, zoo- plankton grazing, death, and sinking. Zooplankton was limited to phy- toplankton feeders, and the zooplankton balance took nutrition, respiration, excretion and death into account. Detritus included dead phyto- and zooplankton and mineralised faeces. The mass balance of dissolved oxygen included deep-sea processes, consumption of oxygen for bottom sediment mineralisation, and exchange across the air-sea interface. The ProDeMo model parameterises light penetration into deep water in relation to phyto- plankton and detritus concentrations (Ołdakowski et al. 2005, this volume). Carried out for the year 2000, the model simulations tookthe water entering the Baltic Sea from the 125 largest rivers into account. For the Vistula (Wisła), the largest river entering the Gulf of Gdańsk, the data in- cluded daily flow-rates and temperatures, and twice-monthly concentrations of nitrates, ammonia, total nitrogen, total phosphorus and dissolved oxygen. Daily concentrations of these nutrients were obtained by interpolation. The daily flow-rates and temperatures for the other rivers were calculated from multi-annual trigonometric series describing the seasonal variability
Details
-
File Typepdf
-
Upload Time-
-
Content LanguagesEnglish
-
Upload UserAnonymous/Not logged-in
-
File Pages24 Page
-
File Size-