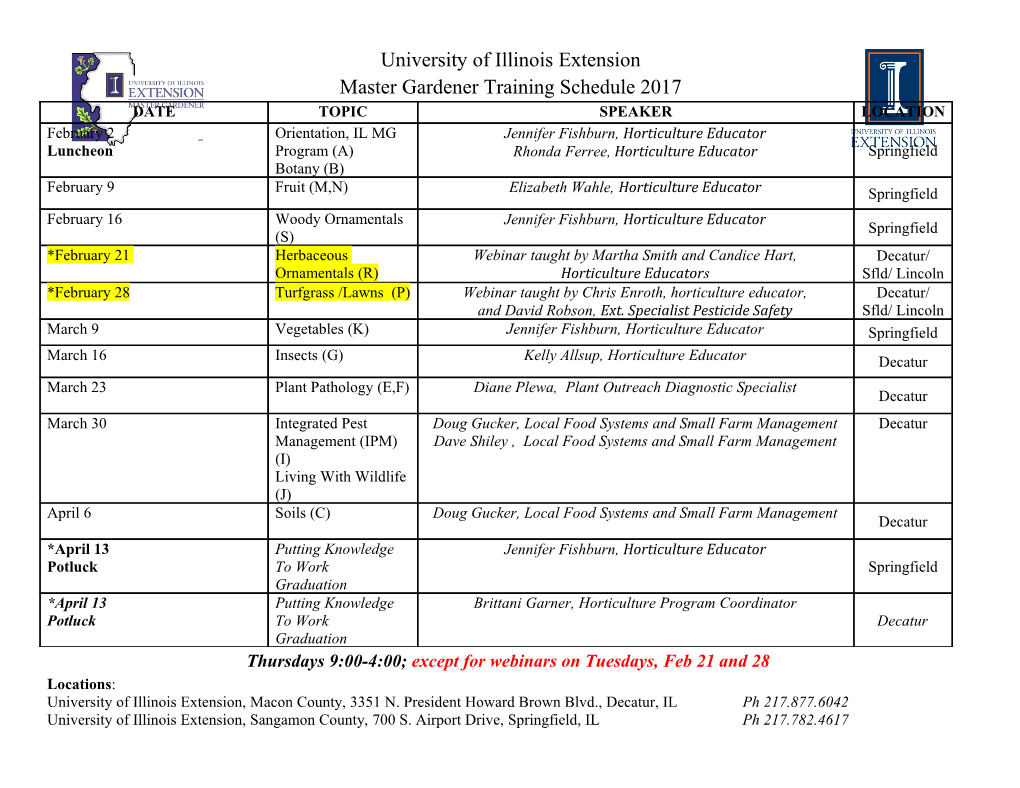
INSTITUTE OF PHYSICS PUBLISHING JOURNAL OF PHYSICS: CONDENSED MATTER J. Phys.: Condens. Matter 17 (2005) R705–R774 doi:10.1088/0953-8984/17/28/R01 TOPICAL REVIEW Electrowetting: from basics to applications Frieder Mugele1,3 and Jean-Christophe Baret1,2 1 University of Twente, Faculty of Science and Technology, Physics of Complex Fluids, PO Box 217, 7500 AE Enschede, The Netherlands 2 Philips Research Laboratories Eindhoven, Health Care Devices and Instrumentation, WAG01, Prof. Holstlaan 4, 5656 AA Eindhoven, The Netherlands E-mail: [email protected] Received 11 April 2005, in final form 10 May 2005 Published 1 July 2005 Online at stacks.iop.org/JPhysCM/17/R705 Abstract Electrowetting has become one of the most widely used tools for manipulating tiny amounts of liquids on surfaces. Applications range from ‘lab-on-a-chip’ devices to adjustable lenses and new kinds of electronic displays. In the present article, we review the recent progress inthis rapidly growing field including both fundamental and applied aspects. We compare the various approaches used to derive the basic electrowetting equation, which has been shown to be very reliable as long as the applied voltage is not too high. We discuss in detail the origin of the electrostatic forces that induce both contact angle reduction and the motion of entire droplets. We examine the limitations of the electrowetting equation and present a variety of recent extensions to the theory that account for distortions of the liquid surface due to local electric fields, for the finite penetration depth of electric fields into the liquid, as well as for finite conductivity effects in the presence of AC voltage. The most prominent failure of the electrowetting equation, namely the saturation of the contact angle at high voltage, is discussed in a separate section. Recent work in this direction indicates that a variety of distinct physical effects—rather than a unique one— are responsible for the saturation phenomenon, depending on experimental details. In the presence of suitable electrode patterns or topographic structures on the substrate surface, variations of the contact angle can give rise not only to continuous changes of the droplet shape, but also to discontinuous morphological transitions between distinct liquid morphologies. The dynamics of electrowetting are discussed briefly. Finally, we give an overview of recent work aimed at commercial applications, in particular in the fields of adjustable lenses, display technology, fibre optics, and biotechnology-related microfluidic devices. (Some figures in this article are in colour only in the electronic version) 3 Author to whom any correspondence should be addressed. 0953-8984/05/280705+70$30.00 © 2005 IOP Publishing Ltd Printed in the UK R705 R706 Topical Review Contents 1. Introduction 706 2. Theoretical background 708 2.1. Basic aspects of wetting 708 2.2. Electrowetting theory for homogeneous substrates 710 2.3. Extensions of the classical electrowetting theory 715 3. Materials properties 719 4. Contact angle saturation 722 5. Complex surfaces and droplet morphologies 725 5.1. Morphological transitions on structured surfaces 725 5.2. Patterned electrodes 726 5.3. Topographically patterned surfaces 727 5.4. Self-excited oscillatory morphological transitions 730 5.5. Electrostatic stabilization of complex morphologies 731 5.6. Competitive wetting of two immiscible liquids 732 6. Dynamic aspects of electrowetting 732 7. Applications 734 7.1. Lab-on-a-chip 734 7.2. Optical applications 737 7.3. Miscellaneous applications 741 8. Conclusions and outlook 741 Acknowledgments 742 Appendix A. Relationships between electrical and capillary phenomena 743 A.1. First law 744 A.2. Second law 749 A.3. Mathematical theory 754 A.4. Electrocapillary motor 757 A.5. Measurement of the capillary constant of mercury in a conducting liquid 760 A.6. Capillary electrometer 762 A.7. Theory of the whirls discovered by Gerboin 766 References 770 1. Introduction Miniaturization has been a technological trend for several decades. What started out initially in the microelectronics industry long ago reached thearea of mechanical engineering, including fluid mechanics. Reducing size has been shown to allow for integration and automation of many processes on a single device giving rise to a tremendous performance increase, e.g. in terms of precision, throughput,and functionality.One prominent example from the area of fluid mechanics the ‘lab-on-a-chip’ systems for applications such as DNA and protein analysis, and biomedical diagnostics [1–3]. Most of the devices developed so far are based on continuous flow through closed channels that are either etched into hard solids such as silicon and glass, or replicated from a hard master into a soft polymeric matrix. Recently, devices based on the manipulation of individual droplets with volumes in the range of nanolitres or less have attracted increasing attention [4–10]. From a fundamental perspective the most important consequence of miniaturization is a tremendous increase in the surface-to-volume ratio, which makes the control of surfaces and surface energies one of the most important challenges both in microtechnology in general as Topical Review R707 well as in microfluidics. For liquid droplets of submillimetre dimensions, capillary forces dominate [11, 12]. The control of interfacial energies has therefore become an important strategy for manipulating droplets at surfaces [13–17]. Both liquid–vapour and solid–liquid interfaces have been influenced in order to controldroplets, as recently reviewed by Darhuber and Troian [15]. Temperature gradients as well as gradients in the concentration of surfactants across droplets give rise to gradients in interfacial energies, mainly at the liquid–vapour interface, and thus produce forces that can propel droplets making use of the thermocapillary and Marangoni effects. Chemical and topographical structuring of surfaces has received even more attention. Compared to local heating, both of these two approaches offer much finer control of the equilibrium morphology. The local wettability andthe substrate topography together provide boundary conditions within which the droplets adjust their morphology to reach the most energetically favourable configuration. For complex surface patterns, however, this is not always possible as several metastable morphologies may exist. This can lead to rather abrupt changes in the droplet shape, so-called morphological transitions, when the liquid is forced to switch from one family of morphologies to another by varying a control parameter, such as the wettability or the liquid volume [13, 16, 18–20]. The main disadvantage of chemical and topographical patterns is their static nature, which prevents active control of the liquids. Considerable work has been devoted to the development of surfaces with controllable wettability—typically coated with self-assembled monolayers. Notwithstanding some progress, the degree of switchability, the switching speed, the long term reliability, and the compatibility with variable environments that have been achieved so far are not suitable for most practical applications. In contrast, electrowetting (EW) has proven very successful in all these respects: contact angle variations of several tens of degrees are routinely achieved. Switching speeds are limited (typically to several milliseconds) by the hydrodynamic response of the droplet rather than the actual switching of the equilibrium valueofthe contact angle. Hundreds of thousands of switching cycles were performed in long term stability tests without noticeable degradation [21, 22]. Nowadays, droplets can be moved along freely programmable paths on surfaces; they can be split, merged, and mixed with a high degree of flexibility. Most of these results were achieved within the past five years by a steadily growing community of researchers in the field4. Electrocapillarity, the basis of modern electrowetting, was first described in detail in 1875 by Gabriel Lippmann [23]. This ingenious physicist, who won the Nobel prize in 1908 for the discovery of the first colour photography method, found that the capillary depression of mercury in contact with electrolyte solutions could be varied by applying avoltage between the mercury and electrolyte. He not only formulated a theory of the electrocapillary effect but also developed several applications, including a very sensitive electrometer and a motor based on his observations. In order to make his fascinating work, which has only been available inFrenchuptonow,availabletoabroader readership, we included a translation of his work in the appendix of this review. The work of Lippmann and of those who followed him in the following more than a hundred years was devoted to aqueous electrolytes in direct contact with mercury surfaces or mercury droplets in contact with insulators. A major obstacle to broader applications was electrolytic decomposition of water upon applying voltages beyond a few hundred millivolts. The recent developments were initiated by Berge [24]inthe early 1990s, who introduced the idea of using a thin insulating layer to separate the conductive liquid from the metallic electrode in order to eliminate the 4 The number of publications on electrowetting has been increasing from less than five per year before 2000 to 8 in 2000, 9 (2001), 10 (2002), 25 (2003), and 34 (2004). R708 Topical Review σ lv σ sv θ σ Y sl U ε d, d Figure 1. Generic electrowetting set-up. Partially wetting liquid droplet at zero voltage (dashed)
Details
-
File Typepdf
-
Upload Time-
-
Content LanguagesEnglish
-
Upload UserAnonymous/Not logged-in
-
File Pages70 Page
-
File Size-