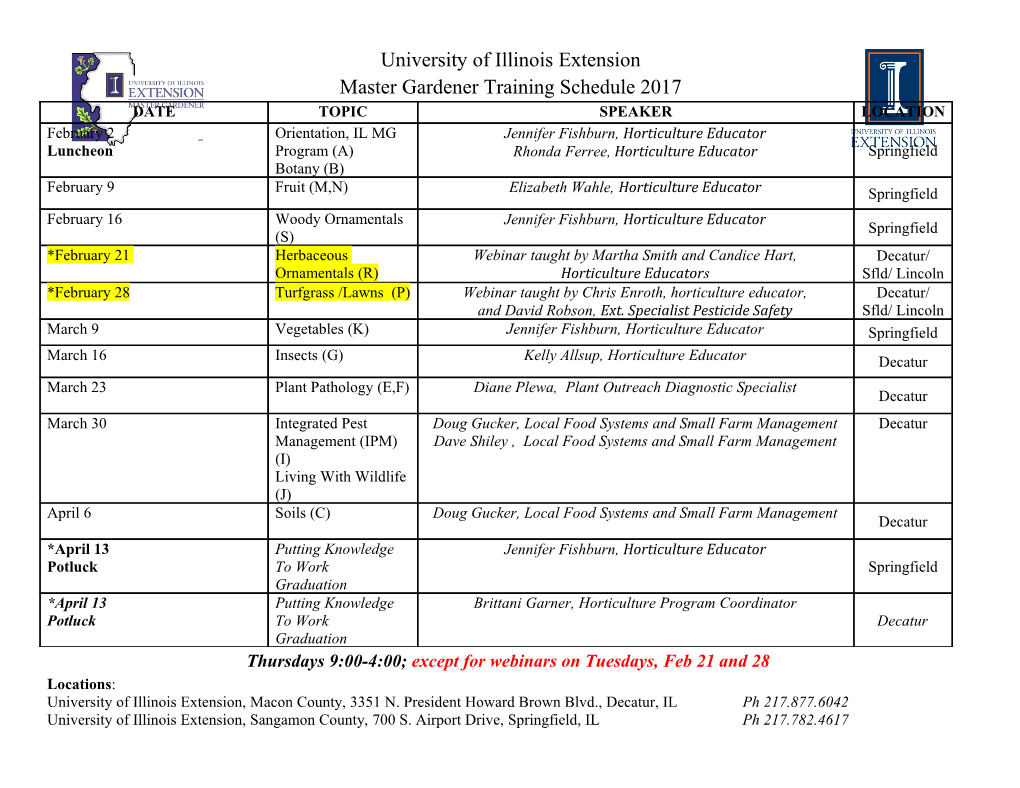
Conners, Shannon Burns. Carbohydrate utilization pathway analysis in the hyperthermophile Thermotoga maritima. (Under the direction of Robert Kelly) Carbohydrate utilization and production pathways identified in Thermotoga species likely contribute to their ubiquity in hydrothermal environments. Many carbohydrate-active enzymes from Thermotoga maritima have been characterized biochemically; however, sugar uptake systems and regulatory mechanisms that control them have not been well defined. Transcriptional data from cDNA microarrays were examined using mixed effects statistical models to predict candidate sugar substrates for ABC (ATP-binding cassette) transporters in T. maritima. Genes encoding proteins previously annotated as oligopeptide/dipeptide ABC transporters responded transcriptionally to various carbohydrates. This finding was consistent with protein sequence comparisons that revealed closer relationships to archaeal sugar transporters than to bacterial peptide transporters. In many cases, glycosyl hydrolases, co-localized with these transporters, also responded to the same sugars. Putative transcriptional repressors of the LacI, XylR, and DeoR families were likely involved in regulating genomic units for β-1,4-glucan, β- 1,3-glucan, β-1,4-mannan, ribose, and rhamnose metabolism and transport. Carbohydrate utilization pathways in T. maritima may be related to ecological interactions within cell communities. Exopolysaccharide-based biofilms composed primarily of β-linked glucose, with small amounts of mannose and ribose, formed under certain conditions in both pure T. maritima cultures and mixed cultures of T. maritima and M. jannaschii. Further examination of transcriptional differences between biofilm-bound sessile cells and planktonic cells revealed differential expression of β-glucan-specific degradation enzymes, even though maltose, an α-1,4 linked glucose disaccharide, was used as a growth substrate. Higher transcripts of genes encoding iron and sulfur compound transport, iron-sulfur cluster chaperones, and iron-sulfur cluster proteins suggest altered redox environments in biofilm cells. Further direct comparisons between cellobiose and maltose-grown cells suggested that transcription of cellobiose utilization genes is highly sensitive to the presence of cellobiose, or a cellobiose-maltose mixture. Increased transcripts of genes related to polysulfide reductases in cellobiose-grown cells and biofilm cells suggested that T. maritima cells in pure culture biofilms escaped hydrogen inhibition by preferentially reducing sulfur compounds, while cells in mixed culture biofilms form close associations with hydrogen-utilizing methanogens. In addition to probing issues related to the microbial physiology and ecology of T. maritima, this work illustrates the strategic use of DNA microarray-based transcriptional analysis for functional genomics studies. CARBOHYDRATE UTILIZATION PATHWAY ANALYSIS IN THE HYPERTHERMOPHILE THERMOTOGA MARITIMA by SHANNON B. CONNERS A dissertation submitted to the Graduate Faculty of North Carolina State University in partial fulfillment of the requirements for the Degree of Doctor of Philosophy BIOINFORMATICS Raleigh 2005 BIOGRAPHY A native of Rome, NY, Shannon Burns Conners graduated summa cum laude from Mount Holyoke College in South Hadley, MA in 1998 with a B.A. in Biochemistry and a minor in Culture, Health and Science. She entered the Bioinformatics program at North Carolina State University in 2000 after transferring from Duke University, where she spent two years as a graduate student in molecular genetics. She joined the Kelly Lab in 2001 and has had the good fortune to participate in a number of interesting projects, including the work collected in this thesis. ii TABLE OF CONTENTS List of Tables……………………………………………………..………..iv List of Figures……………………………………………………..….…v,vi Introduction……………………………………………………..………....1 Introduction References………………………………………..………....5 Chapter 1: Microbial Biochemistry, Physiology, Ecology and Biotechnology of Hyperthermophilic Thermotoga Species……………..10 Chapter 1 References………………………………………..………........51 Chapter 2: Transcriptional analysis of biofilm formation processes in the anaerobic hyperthermophilic bacterium Thermotoga maritima….112 Chapter 2 References………………………………………..………......137 Chapter 3: An expression-driven approach to the prediction of carbohydrate transport and utilization regulons in the hyperthermophilic bacterium T. maritima……………………………..170 Chapter 3 References………………………………………..………......193 Chapter 4: Transcriptional profiles of cellobiose- and maltose-grown T. maritima cells suggest regulatory strategies optimized for ecologically relevant β-linked glucans………………………………………………..233 Chapter 4 References………………………………………..………......253 Research Contributions…………………………………………………278 iii LIST OF TABLES CHAPTER 1: TABLE 1.1. Expression-based functional genomics studies completed or in progress for T. maritima……………………………………………………………………………….98 TABLE 1.2. Selected T. maritima genes and proteins characterized by functional genomics or biochemisty since 1999…………………………………………………….99 TABLE 1.3. ABC transport systems of T. maritima………………………………..….101 TABLE 1.4. Publications for T. maritima proteins characterized structurally by JCSG and others since 1999………………………………………………………………………..103 CHAPTER 2: TABLE 2.1. Thermotoga maritima ORFs differentially regulated in biofilm…………156 TABLE 2.2. Differential expression of genes in biofilm-bound cells as related to predicted T. maritima operons………………………………………………………….161 CHAPTER 3: TABLE 3.1. Carbon sources used in this study……………………………………..…206 TABLE 3.2. List of predicted or confirmed sugar transport systems of T. maritima….207 TABLE 3.S1. Glycoside hydrolases (confirmed and putative) encoded in the Thermotoga maritima………………………………………………………………………….….….208 TABLE 3.S2. T. maritima ABC transport systems examined in this work…………....210 CHAPTER 4: Table 4.1. Sulfur-dependent changes in T. maritima transcripts during batch growth…263 Table 4.2. Carbohydrate-dependent changes in T. maritima transcripts during batch growth………………………………………………………………………………......264 iv LIST OF FIGURES CHAPTER 1: FIG. 1.1. Figure 2. Genomic diversity across the Thermotogales assessed by comparative genomic hybridization (CGH)………………………………………………………….108 FIG. 1.2. Predicted pathway for the utilization of α- and β-glucan polysaccharides by T. maritima.……………………………………………………………………………….110 FIG. 1.3. Predicted pathway for the utilization of β-mannan polysaccharides by T. maritima. ……………………………………………………………………………....111 CHAPTER 2: FIG. 2.1. Thermotoga maritima growth in 1.5-L continuous culture at 80°C…………165 FIG. 2.2. Thermotoga maritima biofilm formation on (A) nylon mesh and reactor walls during continuous cultivation. …………………………………………………………166 FIG. 2.3. Volcano plot showing differential gene expression in planktonic and biofilm Thermotoga maritima cells grown in chemostat culture at 80°C. ……………………..168 FIG. 2.4. A predicted pathway for iron-sulfur cluster biogenesis in Thermotoga maritima biofilm cells.……………………………………………………………………………169 CHAPTER 3: FIG. 3.1. Loop design used for the study of carbon source utilization of T. maritima in this study.…………………………………………………………………………….…219 FIG. 3.2. Circular representation of the T. maritima genome showing locations of known carbohydrate transport proteins and Opp/Dpp family ABC transporter components.….220 FIG. 3.3A. Pentose-responsive loci of T. maritima………………………………….....221 FIG. 3.3B. Pentose-responsive loci of T. maritima………………………………….....222 FIG. 3.3C. Proposed pathway for xylan and pentose uptake in T. maritima…..…….....223 FIG. 3.4. Representative phylogenetic tree of substrate binding proteins of peptide family transporters from T. maritima………………………………………………..…224 FIG. 3.5A. Expression results for transcripts detected at higher levels on β-linked polysaccharides………………………………………………………………………....225 v FIG. 3.5B. Expression results for transcripts detected at higher levels on β-linked polysaccharides………………………………………………………………………....226 FIG. 3.5C. Expression results for transcripts detected at higher levels on β-linked polysaccharides by T. maritima…………………………………………………….......227 FIG. 3.5D. Proposed pathway for the uptake and utilization of β-linked polysaccharides………………………………………………………………………....228 FIG. 3.6A. β-Xylan and xylose-responsive operons from groups 2 and 3 of Opp/Dpp family transporters…………………………………………………………………...…229 FIG. 3.6B. β-Xylan and xylose-responsive operons from groups 2 and 3 of Opp/Dpp family transporters…………………………………………………………………...…230 FIG. 3.7A. Rhamnose responsive locus containing Opp/Dpp family transporter from group 3 of Opp/Dpp family transporters…………………………………….……….....231 FIG. 3.7B. Proposed pathway for the uptake and utilization of rhamnose by T. maritima………………………………………………………………..…….………....232 CHAPTER 4: FIG. 4.1. Loop designs for batch growth and continuous culture microarray experiments……………………………………………………………………………..274 FIG. 4.2. Least squares mean estimates of treatment effects for cellobiose utilization genes (TM1218-1223) and genes within the locus containing a NADH: polysulfide oxidoreductase (TM0379)………………………………………………………………275 FIG. 4.3. Maltose and cellobiose transport, hydrolysis and catabolic pathways include the glycolytic pathways of T. maritima, including enzymes from the EMP and ED pathways………………………………………………………………………………..276 FIG. 4.4. Organization of the hydrogenase operon of T. maritima…………………….277 vi Introduction to Carbohydrate utilization pathway analysis in the hyperthermophile
Details
-
File Typepdf
-
Upload Time-
-
Content LanguagesEnglish
-
Upload UserAnonymous/Not logged-in
-
File Pages287 Page
-
File Size-