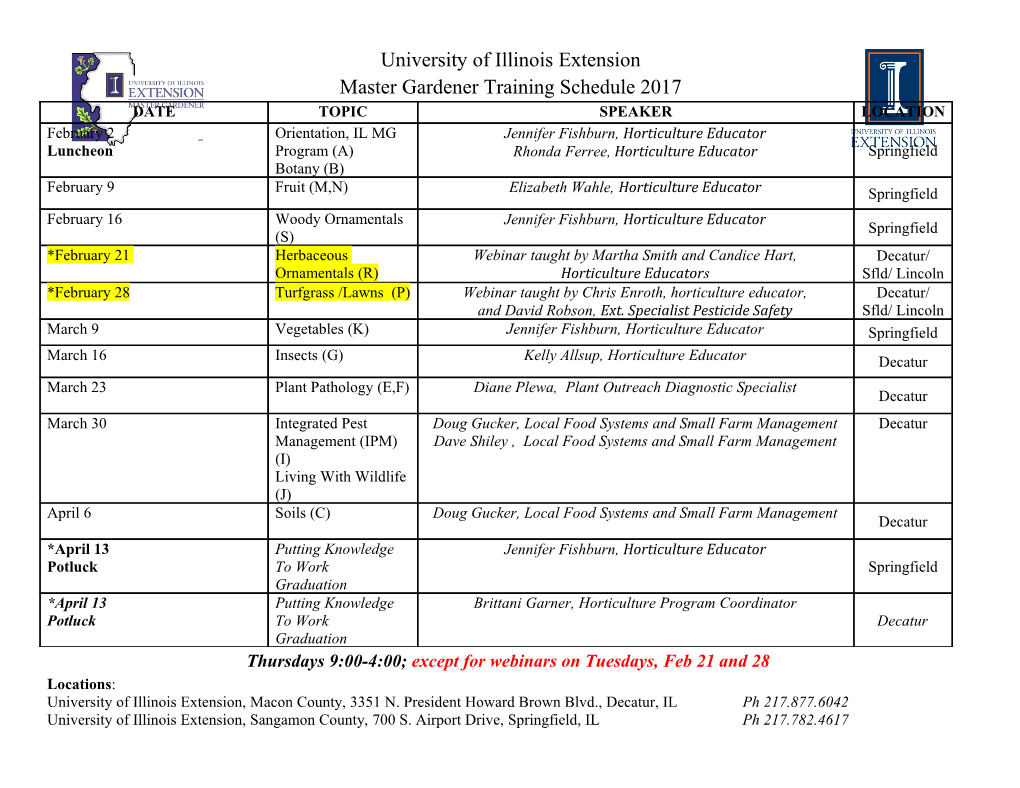
Marisa Mayer Microbial Diversity 2016 Utilizing Squalene Tetrahymanol Cyclase (STC) Degenerate Primer PCR and IMMUNO- FISH to Identify New Microbial Eukaryotes in the Environment BACKGROUND: While there remains a large number of unknowns left to explore across the realm of modern microbiology, few areas remain quite as poorly explored as that of microbial eukaryotes in the environment. Microbial eukaryotes can serve as predators, benefactors, or symbiotic hosts for a number of bacteria and archaea. Most attention to microbial eukaryotes has been based on the medical field and the pathogenicity of certain microbial eukaryotes, while their distribution and biology in their native environments has been largely ignored. These large gaps in the distribution and function of microbial eukaryotes in different ecosystems need to be addressed in order to truly understand the dynamics of different environmental ecosystems. An especially unexplored region of microbiology includes the study of anaerobic eukaryote diversity and function in the environment. This lack of knowledge is partially driven by undersampling of microbial eukaryotes in anaerobic environments and partially driven by the lack of tools developed for identifying microbial eukaryotes in environmental samples. Through my mini project here at the MBL Microbial Diversity course, I hoped to apply degenerate primer PCR, used previously in bacterial environmental samples (Ricci et al., 2014), to identify novel anaerobic eukaryotes. We wanted to use degenerate PCR primers to amplify all known diversity of the squalene tetrahymanol cyclase (stc) gene in our environmental samples. Stc is a gene that cyclizes squalene into tetrahymanol in ciliates such as Tetrahymena (Mallory et al., 1963; Saar et al., 1991). These microbial eukaryote tetrahymanol producers use the tetrahymanol as an analogue for sterols in their cell membrane. Sterols cannot be produced in these organisms because the enzyme needed to cycle squalene into sterols, the oxidosqualene cyclase (osc), cannot function in the absence of sufficient oxygen. Bacteria, also generally lacking sterols in their membranes, have also been found to produce tetrahymanol using a separate pathway (Banta et al., 2015). The bacterial pathway combines the hopanoid producing gene, the squalene hopene cyclase (shc), and a newly discovered gene, the tetrahymanol synthase (ths) gene, to generate tetrahymanol (see Figure 1). squalene hopanoids shc osc sterols Figure 1: Three pathways for sterol or tetrahymanol production in eukaryotes and bacteria. Modified from Banta et al. (2015). Sterols are found in the membranes of organisms across almost all of the eukaryote tree, and thus they are thought to be essential. For this reason, it is no surprise that organisms living in oxygen conditions not conducive to sterol production would develop a pathway to generate a molecule to take over the functions of sterols. Tetrahymanol is a triterpenoid, 5-ringed lipid that does not require oxygen for its biosynthesis. Tetrahymanol is a particularly interesting molecule as its diagenetic product, gammacerane, is a biomarker molecule with implications for past environments in the rock record. Usually tetrahymanol serves as a proxy for oxygen stratification in past ecosystems. It is thought that gammacerane in the rock record stems from ciliates such as tetrahymena and paramecium living in anaerobic conditions who produced the tetrahymanol in place of sterols. Biomarkers are a solution to the detection problem of microbes in the rock record (Eglinton et al., 1964; Eglinton and Calvin, 1967). Biomarkers are the organic molecular remains of living systems such as structurally preserved remains of biomolecules and geochemical remnants of past life. Biomarkers studied range from sterols such as cholesterol (Li et al., 1995), hopanoids such as bacteriohopanepolyols (Summons et al., 1999; Talbot et al., 2003; Waldbauer et al., 2009), or photopigments such as chlorophyll (Jeffrey et al., 1997). Usually in the form of deteriorated lipids, biomarkers are commonly found as fossilized organic matter in petroleum (Ourisson & Albrecht, 1992). These complex, biologically produced molecules are found in rocks that can date back to ~2.5 billion years ago (Brocks et al., 2003). Because of their durability and longevity, biomarker molecules can be used to detect life in ancient sediments dating back to the origins of life on Earth and possibly in deposits on other planetary bodies in the solar system (Simoneit et al., 1998; Summons et al., 2007; Allwood et al., 2013; Jahnke et al., 2014). Many diagnostic remnants of past life can be discovered in ancient sediments and give clues to the nature of microbial life on the early Earth, but these clues are only as good as our interpretation of modern systems. When it comes to microbial eukaryotes, this understanding of their function in modern environments are not understood well enough to decipher the distribution of tetrahymanol in the environment. By using degenerate PCR primers to amplify the squalene tetrahymanol synthase gene in our environmental samples of anaerobic sediments, I hoped to expand our knowledge of anaerobic eukaryotes in samples from Trunk River. Degenerate primers allow us to search for a gene of interest in organisms whose genomes have not been sequenced. I also hoped that the stc primers would find more new sequences of stc than were previously known. The increased number of known anaerobic microbial eukaryotes could then inform a more refined tree of stc genes. This could perhaps help elucidate the evolutionary story of tetrahymanol synthesis in anaerobic eukaryotes. GOALS AND HYPOTHESES: Takishita et al. (2012) previously proposed a tree of the evolutionary relationships between bacterial and eukaryote stc, osc, and shc in which they proposed that the stc gene was laterally transferred from bacteria to the eukaryotes. This tree can be seen in Figure 2. We believe that these interpretations of stc and its evolutionary history are problematic given a) the sparse number of taxa used to construct their tree, b) that the taxa the authors did include in their tree are evolutionary distant from one another, and c) bacteria do not have an stc gene, but instead generate tetrahymanol through a separate pathway which combines the squalene hopene cyclase (shc) gene and the tetrahymanol synthase (ths) gene as described by Banta et al. (2015) and depicted in Figure 1. The notion that key eukaryotic pathways such as the lipid biosynthesis pathways were acquired from horizontal gene transfer has remained in the literature for some time now (Keeling, 2009; Tomazic et al., 2014). In this model, the genes for these processes are not a part of the evolution of the eukaryotes, but were acquired separately from a transfer event somewhere along the line of evolutionary time. Not through vertical inheritance as normal genes are transferred. However, in this instance, it is hard to imagine and seemingly unparsimonious that such an essential gene that produces the only sterol functional equivalent in anaerobic organisms could have been acquired by a chance transfer. Even more hard to imagine, is how such evolutionarily distant eukaryotes could have all acquired this same gene transfer multiple times. These difficulties in conceptualization, in conjunction with the lack of taxa used to construct the Takishita et al. (2012) tree, are why we hypothesized that the squalene tetrahymanol cyclase gene (stc) arose as an early, novel pathway in anaerobic eukaryotes. Furthermore, we hypothesized that finding more anaerobic eukaryote stc sequences would help evolutionary trees of this gene reflect this relationship. My goal for this mini project was to become comfortable with molecular techniques of environmental exploration. In particular, I wanted to learn the skills of degenerate primer construction, PCR methods of probing environmental sample DNA for genes of interest, and the theory behind constructing better phylogenies that can depict more accurate evolutionary relationships between groups of organisms for later hypothesis testing. I also was interested in adding to the amount of data out there on anaerobic eukaryotes as the current amount of knowledge is rather scarce. Figure 2: A tree of lipid biosynthesis genes OSC, STC, and SHC and their proposed evolutionary relationships in bacteria and eukaryotes. From Takishita et al. (2012). METHODS: Environmental Sampling and DNA Extractions: To collect low oxygen environmental samples, we took sediment cores in Trunk River Pond. Figure 3 illustrates the core intact with the various layers marked. We hoped to capture a gradient of oxygen concentrations from the bottom water column to the deep sediments. The cores were roughly 30 cm in depth. water organics organics and sand sand middle Bottom organics and sand Figure 3: Trunk River Pond sediment core sample with sample layers delineated. Before segmenting the core into various layers of interest for downstream analysis, oxygen concentrations were measured by microelectrode probe (see Figure 4). The oxygen concentrations were combined with visual color-based boundary zones in the core to determine the different depths at which sample distinctions should be separated into. The samples were then separated and prepared for DNA extraction of the 5 sediment layers: top oxygenated water, top decaying organics, mixed organics and sand, middle sand, and bottom mixed organics and sand. Eukaryote DNA in the environmental samples was extracted using
Details
-
File Typepdf
-
Upload Time-
-
Content LanguagesEnglish
-
Upload UserAnonymous/Not logged-in
-
File Pages12 Page
-
File Size-