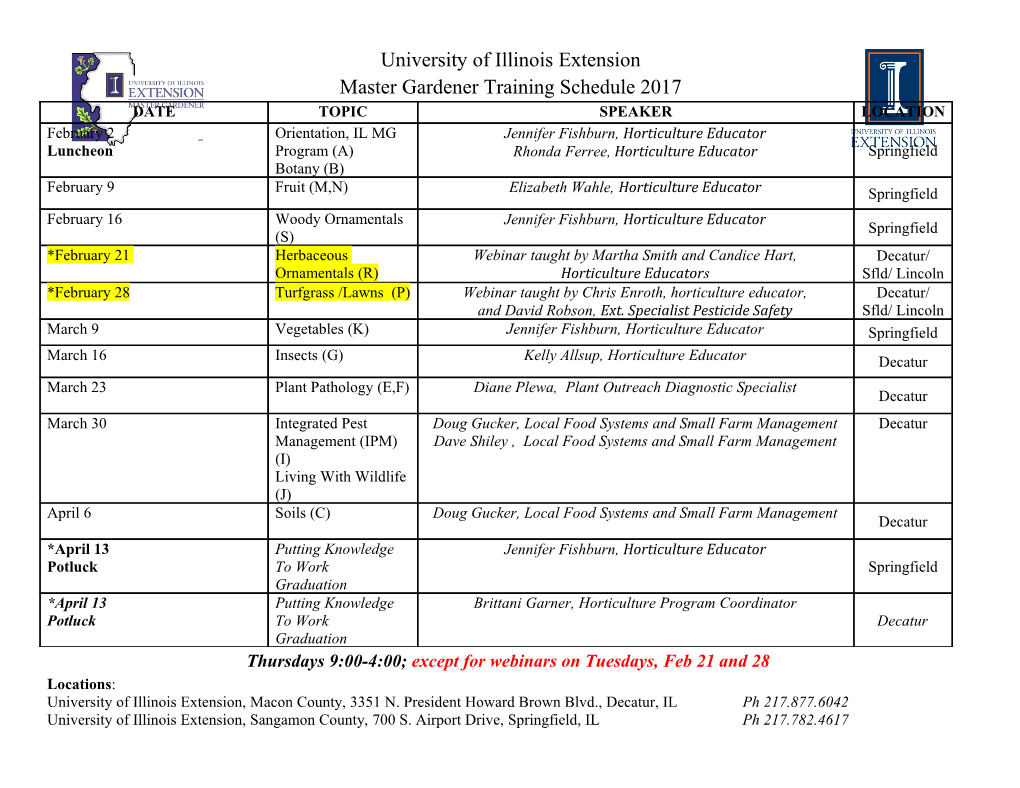
Mechanical Quantum Systems Matt LaHaye – Syracuse University Supported by: NSF-DMR Career Award: #1056423; NSF-DMR Materials World Network Award: #1312421 Paraty Quantum Information and Computation 2015 LaHaye Research Group at Syracuse Postdoc: Dr. Francisco Rouxinol (UNICAMP) Microwave electronics Graduate Student: Hugo Hao Helium liquefier Dilution Refrigerator - milli-Kelvin Undergraduate: Theory Collaborators: Profs. Fred Brito temperatures Andrew Ballard (Sao Carlos)& Amir Caldeira (UNICAMP) Mechanical Quantum Systems (MQS) Develop and study mechanical quantum systems; systems which under ordinary conditions are perfectly well-described by classical laws of physics Graphene Sheets Micro-toroid Structures Devices like: Steele (Delft) Nanomechanical Beams Roukes (Caltech) Kippenberg (EPFL) Superfluid Acoustic Cavities Schwab (Caltech) Just a small subset of types of devices being explored. LIGO Macroscopic Mirrors Mechanical Quantum Systems (MQS) The motional properties of these ‘everyday’ structures are normally well- represented in terms of classical harmonic oscillator modes Devices like: Microtoroid Resonators Nanomechanical Beams Roukes (Caltech) Kippenberg (EPFL) But… - Well-defined and that Vibrational modes ‘ring’ as follow Netwon’s second law one would expect for a - Continuous energy spectrum classical simple harmonic oscillator - No superposition/interference Mechanical Systems in the Quantum Regime No reason that we know of why the motion of such objects shouldn’t exhibit characteristics of quantum S.H.O. (under the right conditions) Cantilever in a quantum superposition Roukes (Caltech) of spatially-separated states From Schwab & Roukes, Phys. Today 2005 1 = ( + ) 2 k ℏ Discrete Energy m x Levels Zero-point Early Ideas See: M.L. Roukes, Physics World 14, 25 (2001); and V. Braginsky & F. Khalili, Quantum Measurement fluctuations Mechanical Quantum Systems: Motivations and Applications Fundamental studies of quantum mechanics - Test decoherence in new limits; perform Schrodinger-Cat experiment with devices we normally observe to have definite properties; etc - Further our understanding of fundamental limits of measurement Development of new technologies Long-distance quantum communication - For quantum information e.g. Optomechanical transducers See: J.T. Hill et al. Nat. Comm. (2012) J. Bochmann et al. Nat. Phys. (2013) T. Bagci et al. Nature (2014) K. Stannigel et al. PRA 2011 R. Andrews et al. Nat. Phys. (2014) e.g. - For quantum sensing and imaging - For gravitational wave detection - Energy dissipation at nanoscale Single-Nuclei Magnetic Imaging Rugar et al. Nature 2004 Recommended Reviews on the MQS Field Broader Audience Markus Aspelmeyer, Pierre Meystre & Keith Schwab Physics Today 65, 29 (2012) Some Technical Reviews Keith Schwab, Michael Roukes - M. Poot & H.S. van der Zant. Phys. Rep. 511, 273 (2012). Physics Today 58, 36 (2005) - Y. Greenberg et al. Physics –Uspekhi 55, 382 (2012). - M. Aspelmeyer et al. Rev. Mod. Phys. 86, 1391 (2014). - G. Kurizki et al. Proc. Natl. Acad. Sci. 112, 3866 (2015). Outline of the Lectures Lecture 1: Origins/Motivations of MQS Confluence of several paths of research From gravitational wave detection to nanomechanics Lecture 2: Exploring Quantum Limits of Mechanics Basic criteria for observing quantum behavior in other- wise classically-behaving systems State of the art in regard to meeting these basic criteria Lecture 3: Superconducting qubits and mechanics A mechanical analog to CQED Great promise to serve as a test-bed for studying MQS STEP BACK AND LOOK AT THE ORIGINS AND MOTIVATIONS Nanomechanics Mesoscopic Quantum Devices & Superconducting Devices nano.caltech.edu Primary Influences on the lahayelab.syr.edu Development of Mechanical Gravitational Wave Detection Quantum Systems Quantum Optics & CQED www2.cnrs.fr/en/1460.htm gravity.phy.syr.edu Origins: Gravitational Wave Detection References: P.R. Saulson, Physics of gravitational wave detection… XXVI SLAC Summer Institute on Particle Physics (1998). Artist rendering of the Gravitational Waves coalescence of two black holes - Prediction of general relativity - Traveling space-time oscillations that propagate at the speed of light - So far, not directly observed… In principle could be observed by measuring changes in relative http://silkroad.bao.ac.cn/web/ spacing of objects as wave passes Example1. Two black holes coalescing; each Black hole being 10 solar masses. + ( ) The gravitational signal measured at a distance of 200 Mpc is ∆ Before wave During wave = 5 × 10 ! - However, such oscillations are for controlled∆ (i.e. laboratory-based)−21 GW incredibly small and are likely only be signalsℎ would be⁄ orders≈ of magnitude smaller! observable for astrophysical∆ events ℎ Origins: Gravitational Wave Detection References: P.R. Saulson, Physics of gravitational wave detection… XXVI SLAC Summer Institute on Particle Physics (1998). Artist rendering of the Gravitational Waves coalescence of two black holes - Prediction of general relativity - Traveling space-time oscillations that propagate at the speed of light - So far, not directly observed… In principle could be observed by measuring changes in relative http://silkroad.bao.ac.cn/web/ Raisesspacing ofthe objects Question: as wave passes How can one detect such small strains? Example1. Two black holes coalescing; each Black hole being 10 solar masses. + ( ) The gravitational signal measured at a distance of 200 Mpc is ∆ Before wave During wave = 5 × 10 ! ∆ −21 - Importance of detection: confirmation of predictions of GR; novel studies of “hard to see” astrophysical phenomena; new era of gravitationalℎ wave⁄ astronomy≈ Origins: Gravitational Wave Detectors (Resonant Bars) Reference: K. Riles, Progress in Particle & Nuclear Physics 68 (2013). Resonant Mass Detectors Massive superconducting bars (~ 2 Tons) GW “rings up” lowest mechanical mode (Q ~ 10 ) 6 Resonant transducer amplifies motion and converts to electrical domain for read-out with sensitive electronics Aluminum 5056 Source: auriga.lnl.infn.it (~1 kHz) Origins: Gravitational Wave Detectors (Resonant Bars) Reference: K. Riles, Progress in Particle & Nuclear Physics 68 (2013). Source: auriga.lnl.infn.it Joseph Weber, circa 1965. - Original “Weber bars” used piezoelectric transducers as strain sensors - Not cryogenic, limited by RT thermal noise to strain sensitivity of ~10 State of the art today: −16 - 3 m bar cooled to 100’s mK. SQUIDs used in electronic read-out ℎ - Strain sensitivities ~ 10 achieved (Corresponds to displacement sensitivity ~ 10 m! ). −21 −21 ℎ Origins: Gravitational Wave Detectors (Resonant Bars) References: K. Riles, Progress in Particle & Nuclear Physics 68 (2013). Source: auriga.lnl.infn.it No confirmed GW detection events yet… Limitation: They are resonant devices, so operation limited to narrow bandwidth (~10-100 Hz) around 1 kHz Limits range of GW sources it can “see” See Riles (above) and B.S. Sathyaprakash.→ Living Rev. Relativity 12, 2 (2009) for more details about state of the art. Joseph Weber, circa 1965. - Original “Weber bars” used piezoelectric transducers as strain sensors - Not cryogenic, limited by RT thermal noise to strain sensitivity of ~10 State of the art today: −17 - 3 m bar cooled to 100’s mK. SQUIDs used in electronic read-out ℎ - Strain sensitivities ~ 10 achieved (Corresponds to displacement sensitivity ~ 10 m! ). −21 −21 ℎ Origins: Gravitational Wave Detectors (Interferometers) References: K. Riles, Progress in Particle & Nuclear Physics 68 (2013). Incident GW Hanford, WA USA 4 km long arms! Source: LIGO (Laser Interferometer Gravitational Wave Observatory) Livingston, LA USA Source: ligo.caltech.edu - Incident GW changes path lengths light in the Michelson interferometery’s arms - Leads to a phase difference in light returning from each arm to the beam splitter Source: LIGO Collaboration - Phase difference is proportional to arm length Strain sensitivity 1/ → ∝ Origins: Gravitational Wave Detectors (Interferometers) References: K. Riles, Progress in Particle & Nuclear Physics 68 (2013). Hanford, WA USA 4 km long arms! Source: LIGO (Laser Interferometer Gravitational Wave Observatory) Source: Riles. Livingston, LA USA - LIGO Hanford and Livingston (H1 & L1) and Virgo (V1) have achieved 10 over broad band (10’s Hz to kHz)! −21 ℎ ≤ - No detection events yet, but new upgraded run (Advanced LIGO) has begun Source: LIGO Collaboration with factor of 10 improvement expected Origins: Gravitational Wave Detectors (Interferometers) References: K. Riles, Progress in Particle & Nuclear Physics 68 (2013). Hanford, WA USA 4 km long arms! Both resonant mass detectors and Km-scale interferometers perform Source: LIGOmeasurements (Laser Interferometer of displacement Gravitational with sensitivity orders-of-magnitude smaller Wave Observatory)than the width of atomic nuclei… Source: Riles. This raises someLivingston, important LA USA questions - LIGO Hanford and Livingston (H1 & L1) and Virgo (V1) have achieved 10 over broad band (10’s Hz to kHz)! −21 ℎ ≤ - No detection events yet, but new upgraded run (Advanced LIGO) has begun Source: LIGO Collaboration with factor of 10 improvement expected Origins: Gravitational Wave Detection – Questions Raised About Position Measurement Is it sensible to talk about measuring displacements of macroscopic bodies that are a small fraction of an atomic nucleus? (yes!) Does quantum mechanics come into play at all? (yes!) Subject Zero-point fluctuations Discussed In Lecture II Quantum noise and back-action If so, does quantum mechanics
Details
-
File Typepdf
-
Upload Time-
-
Content LanguagesEnglish
-
Upload UserAnonymous/Not logged-in
-
File Pages50 Page
-
File Size-