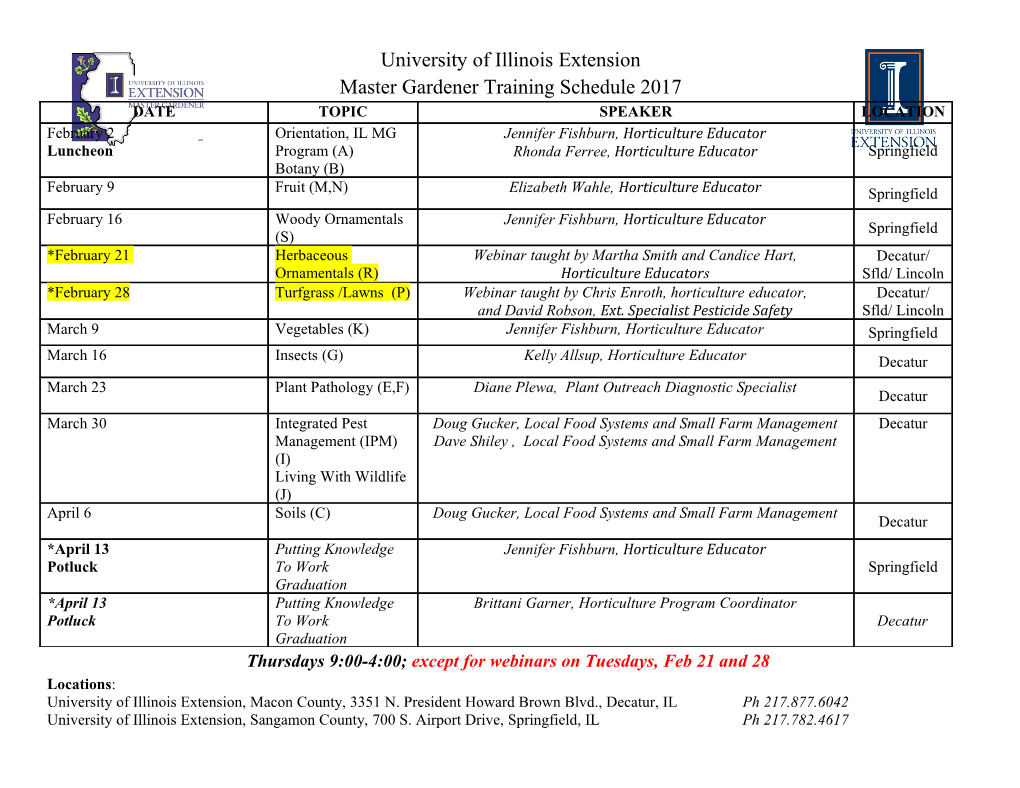
LETTER doi:10.1038/nature13795 Identification of an iridium-containing compound with a formal oxidation state of IX Guanjun Wang1, Mingfei Zhou1, James T. Goettel2, Gary J. Schrobilgen2, Jing Su3, Jun Li3, Tobias Schlo¨der4 & Sebastian Riedel4,5 One of the most important classifications in chemistry and within the indicating that more than one isomer is experimentally observed, be- 1–4 1 periodic table is the concept of formal oxidation states .Theprepa- cause any [IrO4] structure should at most have four vibrational fun- ration and characterization of compounds containing elements with damentals in the Ir5O and O–O stretching regions. Experiments with unusual oxidation states is of great interest to chemists5. The highest different time delays between expansion from the pulsed valve and vapor- experimentally known formal oxidation state of any chemical ele- ization (Methods), and with different stagnation pressures, suggest that ment is at present VIII2–4, although higher oxidation states have been the bands at 936 and 944 cm–1 are due to the same species, whereas the postulated6,7. Compounds with oxidation state VIII include several other three absorptions are caused by other isomers. All bands were shifted 8 18 xenon compounds (for example XeO4 and XeO3F2) and the well- to lower wavenumbers in the experiments using O2 (Supplementary 16 18 characterized species RuO4 and OsO4 (refs 2–4). Iridium, which has Information), and the observed frequency shifts with O/ O isotopic nine valence electrons, is predicted to have the greatest chance of being ratios in the range of 1.050–1.056 suggest that these bands originate from oxidized beyond the VIII oxidation state1. In recent matrix-isolation Ir5OorO2O stretching vibrations. experiments, the IrO4 molecule was characterized as an isolated mole- Recent quantum-chemical calculations show that three low-energy 1 10 cule in rare-gas matrices9. The valence electron configuration of iridium isomers are possible for a cation of [IrO4] stoichiometry .Thesecations 1 in IrO4 is 5d , with a formal oxidation state of VIII. Removal of the have been reinvestigated by more accurate ab initio coupled-cluster cal- remaining d electron from IrO4 would lead to the iridium tetroxide culations with single and double excitations and perturbative-triples 1 10 corrections (CCSD(T)), as wellas by ab initio multi-reference-based com- cation ([IrO4] ), which was recently predicted to be stable and in which iridium is in a formal oxidation state of IX. There has been some plete active space perturbation theory (CASPT2) calculations (Methods). 1 11,12 On the basis of these calculations, the wavenumber of the O2O stretch- speculation about the formation of [IrO4] species , but these expe- –1 rimental observations have not been structurally confirmed. Here ing mode of the superoxide complex is predicted to be 1,486.3 cm at 1 density functional level with inclusion of dispersion corrections (B3LYP- we report the formation of [IrO4] and its identification by infrared 21 photodissociation spectroscopy. Quantum-chemical calculations were D3) or 1,458.7 cm (CCSD(T)) with appreciable infrared intensity. 1 VI 1 3 carried out at the highest level of theory that is available today, and Hence, this superoxide complex [(g -O2)Ir O2] (Cs symmetry, A99 ground state) can be ruled out because of the absence of any observed predict that the iridium tetroxide cation, with a Td-symmetrical struc- 21 ture and a d0 electron configuration, is the most stable of all possible band in the experimental spectra above 1,100 cm . The observed absorp- 2 VII 1 1 tions probably come from the side-on O2 complex [(g -O2)Ir O2] [IrO4] isomers. 1 IX 1 1 Iridium oxide cations were generated in the gas phase using a pulsed- (C2v, A1) and the tetroxide complex [Ir O4] (Td, A1), where the laser vaporization/supersonic-expansion source and were studied by b 2.0 –1 [IrO ]+ infrared photodissociation spectroscopy in the 850–1,600 cm region 4 as described previously13. A typical mass spectrum of the iridium oxide cations produced with O -seeded helium is shown in Fig. 1a. The spec- + +• 2 1.5 [IrO3] [IrO4] Arn trum consists of a progression of peaks having different masses that n = 1 65432 1 Ir+ correspond to different [IrOx] species with up to six oxygen atoms. 1 Enhanced abundance in the mass spectrum was found for [IrO4] , and [IrO ]+ the preferential formation of this cation indicates increased stability. Be- 1.0 2 1 cause the dissociation energies of the [IrO4] cations are significantly a Intensity greater than the infrared photon energies in the Ir5O and O–O stretch- ing frequency region (the infrared photons in the 900–1,200 cm21 region 0.5 have energies in the range of 10.8–14.4 kJ mol21; Supplementary Infor- mation), the method of rare-gas atom predissociation is employed to 14–17 [IrO ]+ obtain the infrared spectra for these molecules . When argon was 6 1 used instead of helium, [IrO4] ?Arn ions (n 5 1, 2 and larger; the dot 0.0 denotes a weak bond) were produced (Fig. 1b) and the mass peaks corre- 150 200 250 300 350 400 450 500 550 193 1 sponding to the [ IrO4] ?Arn isotopomers were selected for photo- m/z dissociation. When the infrared laser is on resonance with one of the 1 Figure 1 | Mass spectra of the iridium oxide cations. The cations are vibrational fundamentals of an [IrO ] ?Ar complex, the latter photo- 4 n produced by pulsed-laser vaporization of an iridium metal target in an dissociates by eliminating an argon atom. The resulting predissociation 1 expansion of helium (a) or argon (b) seeded by dioxygen. The isotopic splitting infrared spectra of [IrO4] are shown in Fig. 2. of iridium can clearly be resolved with the relative peak areas matching the 1 191 193 The experimental spectrum of [IrO4] ?Ar (Fig. 2a) consists of five ab- natural abundance isotopic distribution ( Ir, 37.3%; Ir, 62.7%). m/z, mass/ sorptions at 936, 944, 966, 1,047 and 1,054 cm21,respectively,(Table1), charge ratio; intensity is shown in arbitrary units. 1Collaborative Innovation Center of Chemistry for Energy Materials, Department of Chemistry, Shanghai Key Laboratory of Molecular Catalysts and Innovative Materials, Fudan University, Shanghai 200433, China. 2Department of Chemistry, McMaster University, 1280 Main Street West, Hamilton, Ontario L8S 4M1, Canada. 3Department of Chemistry and Key Laboratory of Organic Optoelectronics and Molecular Engineering of Ministry of Education, Tsinghua University, Beijing 100084, China. 4Institut fu¨r Anorganische und Analytische Chemie, Albert-Ludwigs Universita¨t Freiburg, Albertstrasse 21, D-79104 Freiburg im Breisgau, Germany. 5Institut fu¨ r Chemie und Biochemie – Anorganische Chemie, Freie Universita¨t Berlin, Fabeckstrasse 34-36, D-14195 Berlin, Germany. 23 OCTOBER 2014 | VOL 514 | NATURE | 475 ©2014 Macmillan Publishers Limited. All rights reserved RESEARCH LETTER a 12 200 190 8 150 4 ) –1 122.1 0 100 167.9 b (kJ mol 81 E 8 Δ 209.6 50 40 (89) 4 170.8 51 (129) 141.2 0 0 0 188.8 168.0 c Intensity 8 + [(η2-O )IrO ]+ [(η1-O )IrO ]+ [IrO4] 2 2 2 2 4 Figure 3 | Optimized structures and energetic ordering of the different 1 [IrO4] isomers. The structures are optimized at the CCSD(T) level. The barriers of the interconversion reactions are also shown. The relative energies 0 are calculated at the SO-DFT/B3LYP and CCSD(T) (in parentheses) levels, 1 d with the energy of the most stable isomer ([IrO4] ) set to zero. The symmetries 1 1 2 1 1 8 and ground states are as follows: [IrO4] (Td, A1), [(g -O2)IrO2] (C2v, A1), 1 1 3 –1 [(g -O2)IrO2] (Cs, A0). Bond lengths are in pm; energies are in kJ mol . Red, oxygen atoms; grey, iridium atoms. 4 1 The second most stable isomer of [IrO4] stoichiometry is the C2v- 2 VII 1 symmetrical side-on complex [(g -O2)Ir O2] , which was also calcu- 0 lated to have a closed-shell electron configuration. All three isomers were 900 950 1,000 1,050 1,100 calculated to be true minima on the potential energy surface and are en- Wavenumber (cm–1) ergetically separated by 89 and 40 kJ mol–1, respectively, at the CCSD(T) 193 1 Figure 2 | Infrared photodissociation spectra of the [ IrO4] ?Arn level (Fig. 3). Our calculations show that their energetic ordering is not (n 5 1–4) cations. The spectra are measured by monitoring the Ar affected by weak argon coordination (Supplementary Information). 193 1 193 1 1 1 2 1 photodissociation channel: a,[ IrO4] ?Ar; b,[ IrO4] ?Ar2; The barrier for the [(g -O2)IrO2] R [(g -O2)IrO2] conversion was 193 1 193 1 21 c,[ IrO4] ?Ar3; d,[ IrO4] ?Ar4. Intensity is shown as the yield of calculated to be only about 30 kJ mol using density functional theory fragmentation ions normalized to the parent ion signal in percentage. with relativistic spin–orbit coupling effects (SO-DFT/B3LYP; Fig. 3 and 2 1 Supplementary Information). The barrier for the [(g -O2)IrO2] R 1 –1 latter is by far the most stable isomer according to high-level (coupled- [IrO4] reaction lies at 150 kJ mol at the SO-DFT/B3LYP level (Fig. 3 cluster) calculations (Fig. 3). and Supplementary Information). This barrier is remarkably high, but Following the general rules for the determination of formal oxidation nevertheless is plausible because the isomerization reaction involves the 1 states, the experimentally identified cation [IrO4] can be viewed as an electron transfer and cleavage of the relatively strong peroxide O2O 18 2 1 1 Ir(IX) species. This assignment is in line with the well-known isoelec- bond .
Details
-
File Typepdf
-
Upload Time-
-
Content LanguagesEnglish
-
Upload UserAnonymous/Not logged-in
-
File Pages4 Page
-
File Size-