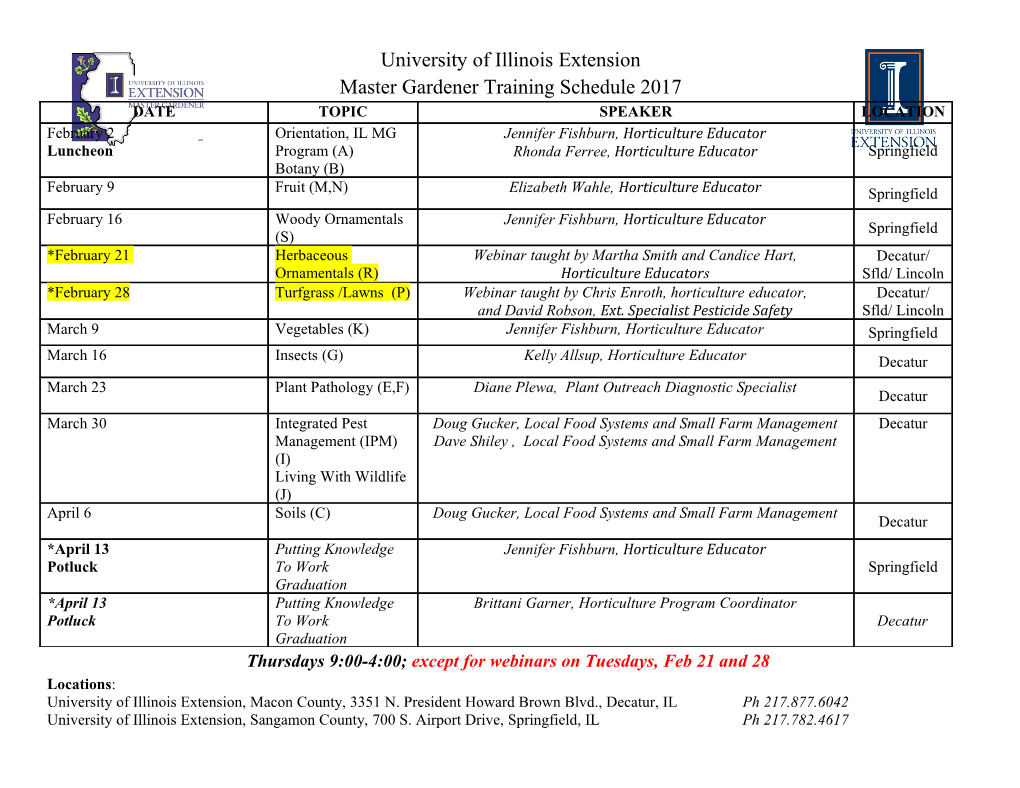
ARTICLE https://doi.org/10.1038/s41467-021-21852-7 OPEN Flexible and high-performance electrochromic devices enabled by self-assembled 2D TiO2/MXene heterostructures Ran Li1,2,7, Xiaoyuan Ma 2,7, Jianmin Li1, Jun Cao 2, Hongze Gao3, Tianshu Li 3, Xiaoyu Zhang1, Lichao Wang1, Qinghong Zhang 1, Gang Wang1, Chengyi Hou 1, Yaogang Li1, Tomás Palacios4, ✉ ✉ ✉ Yuxuan Lin 4,6 , Hongzhi Wang 1 & Xi Ling 2,3,5 1234567890():,; Transition metal oxides (TMOs) are promising electrochromic (EC) materials for applications such as smart windows and displays, yet the challenge still exists to achieve good flexibility, high coloration efficiency and fast response simultaneously. MXenes (e.g. Ti3C2Tx) and their derived TMOs (e.g. 2D TiO2) are good candidates for high-performance and flexible EC devices because of their 2D nature and the possibility of assembling them into loosely networked structures. Here we demonstrate flexible, fast, and high-coloration-efficiency EC devices based on self-assembled 2D TiO2/Ti3C2Tx heterostructures, with the Ti3C2Tx layer as the transparent electrode, and the 2D TiO2 layer as the EC layer. Benefiting from the well- balanced porosity and connectivity of these assembled nanometer-thick heterostructures, they present fast and efficient ion and electron transport, as well as superior mechanical and electrochemical stability. We further demonstrate large-area flexible devices which could potentially be integrated onto curved and flexible surfaces for future ubiquitous electronics. 1 State Key Laboratory for Modification of Chemical Fibers and Polymer Materials, College of Materials Science and Engineering, Donghua University, Shanghai, China. 2 Department of Chemistry, Boston University, Boston, MA, USA. 3 Division of Materials Science and Engineering, Boston University, Boston, MA, USA. 4 Department of Electrical Engineering and Computer Science, Massachusetts Institute of Technology, Cambridge, MA, USA. 5 The Photonics Center, Boston University, Boston, MA, USA. 6Present address: Department of Electrical Engineering and Computer Sciences, University of California, ✉ Berkeley, CA, USA. 7These authors contributed equally: Ran Li, Xiaoyuan Ma. email: [email protected]; [email protected]; [email protected] NATURE COMMUNICATIONS | (2021) 12:1587 | https://doi.org/10.1038/s41467-021-21852-7 | www.nature.com/naturecommunications 1 ARTICLE NATURE COMMUNICATIONS | https://doi.org/10.1038/s41467-021-21852-7 lectrochromic (EC) materials, which can change their the fastest coloration speed, the highest coloration efficiency and optical absorption reversibly due to the electrochemical superior electrochemical stability. We further demonstrate a E 1–3 fl redoxation with inserted ions under an applied potential , large-area exible device which could potentially be integrated have shown great promise in applications such as displays, smart with arbitrary curved and flexible surfaces for future ubiquitous windows and optical camouflage3–5. With the growing interest in electronics. bringing electrochromism to the field of flexible and wearable electronics in recent years, incorporating the functionality of flexibility to EC devices while maintaining high energy efficiency, Results fast switching speed and long durability has become increasingly Preparation of 2D TiO2 via the chemical conversion from 6,7 critical . However, this is an extremely challenging task because: Ti3C2Tx. Figure 1 illustrates the fabrication process of the self- (a) both the EC performance and the mechanical properties are assembled TiO2/Ti3C2Tx heterostructure on a flexible structure as nontrivially correlated with the materials of choice, their nanos- the core functional component of EC devices. The fabrication cale formations, and the assembly approach; and (b) the co- process consists of three steps: First, the Ti3C2Tx nanosheet dis- optimization of the transparent conductive electrode and the EC persion in deionized (DI) water is obtained from selective etching 22 material, as well as the way to integrate them, are needed. of Ti3AlC2 powder (MAX phase) , and diluted to 0.2 mg/L, Recently, two-dimensional (2D) transition metal carbides, followed by the formation of the Ti3C2Tx aerogel through freeze nitrides, and carbonitrides (referred to as MXenes) have emerged drying. The Ti3C2Tx aerogel is then annealed in air at 400 °C to as promising nanoscale electronic materials with good electro- fully be converted to the TiO2 aerogel; Second, the Ti3C2Tx 8,9 chemical stability . They have also been reported as promising (TiO2) aerogels are dispersed into isopropyl alcohol (IPA) to form templates for the synthesis of functional micro/nanostructured the Ti3C2Tx (TiO2) solutions, which are then injected into the materials, such as transition metal oxides (TMOs), alkali-metal interface between hexane and water, where the nanosheets self- titanates, and their hybrids10,11. Owing to their unique optical assemble into uniform nanometer-thick films; Last, the self- fi fi and electrical properties, MXenes and their derivatives have assembled Ti3C2Tx lm and TiO2 lm are transferred sequentially demonstrated their potential in the application of energy storage, onto a flexible polyethylene terephthalate (PET) substrate to form 12–14 fi catalysis, and electronics . More importantly, 2D nanosheets TiO2/Ti3C2Tx heterostructures, in which the Ti3C2Tx lm serves fi of both MXenes and their derivatives can be produced in liquid as the transparent conductive electrode, and the TiO2 lm serves phase scalably, which makes it easy for constructing self- as the EC layer in EC devices (more details about film assembly assembled films and hybrid structures, thus suitable for device and transfer processes are in Supplementary Fig. 1, Supplemen- applications in large scale. tary Movie 1 and Supplementary Movie 2 in Supplementary We propose that self-assembled MXene/TMO hetero- Information). fl structures, with the MXenes as the exible transparent electrode, To obtain the anatase phase of TiO2, which has been fl 23 and the TMOs (derived from the MXenes) as the exible EC demonstrated a good EC material , we anneal the Ti3C2Tx layer, are promising for high performance and flexible EC devices, aerogel in air at 400 °C for 50 h. X-ray diffraction (XRD) and yet it has never been explored previously. In addition to the Raman spectroscopy of the flakes are measured before and after advantage of the easiness of fabrication and integration with the thermal annealing process to investigate the compositional different functional components from MXene derivatives as and the structural changes. As shown in Fig. 2a, after annealing in mentioned above, an even more important reason is that the air at 400 °C, new peaks at 26°, 38°, 48°, 54°, and 63° appear in the nanosheets network of TMOs, such as tungsten oxide (WO3) and XRD spectra, corresponding to the (101), (112), (200), (105), and 24 titanium oxide (TiO2), can potentially realize not only superior (204) planes of the anatase phase of TiO2 . Raman spectra of EC properties, but also reliable mechanical and chemical stability Ti3C2Tx flakes after annealing confirms the conversion −1 among various candidates of EC materials. First, these inorganic from Ti3C2Tx to TiO2 (Fig. 2b), where peaks at 145 cm (Eg), −1 −1 −1 materials are long-lasting and robust against environmental fac- 398 cm (B1g), 518 cm (B1g), and 639 cm (Eg) match well 25 tors such as high temperature, ultraviolet radiation, and with the Raman features of the anatase phase of TiO2 . X-ray mechanical wear15–17, as compared to their organic counter- photoelectron spectroscopy (XPS) characterization further sug- 18 ’ parts . Second, the nanostructured TMO network, especially in gests the transformation from Ti3C2Tx to TiO2.Its clearly seen the form of 2D nanosheets, improves electrical and ionic trans- that the peaks for the Ti element in Ti3C2Tx (i.e., Ti-C 2p3/2 fi port signi cantly, because: (a) the increased surface-to-volume (455.3 eV), Ti(II) 2p3/2 (456.1 eV), Ti(III) 2p3/2 (457.2)) disappear ratio of nanosheets as compared to the bulk forms leads to larger after the annealing (Fig. 2c top and Supplementary Fig. 2), while interface areas with the electrolyte and the shorter ion diffusivity peaks at 458.8 eV and 464.8 eV, corresponding to Ti(IV) 2p3/2 3,15 26 pathways within the nanostructured channels; and (b) the 2D and Ti(IV) 2p1/2 in TiO2, become dominant (Fig. 2c bottom) . formation of the nanostructures makes the contact area between To evaluate the morphology and crystallinity of the obtained adjacent nanosheets much larger than that between 1D or 0D TiO2 nanosheets, optical microscopy (OM), transmission electron structures (nanowires or quantum dots)19, which promotes the microscopy (TEM) and atomic force microscopy (AFM) are used 20 fl internanosheets electron transfer . Finally, networks of 2D to characterize Ti3C2Tx akes before and after the in situ nanostructures also help to redistribute the induced strain evenly, conversion to TiO2. Supplementary Fig. 3 shows OM images of a thus improving both the mechanical strength and the flexibility of typical flake (~7 µm in lateral dimension) undergoing the the film, which is fundamentally more advantageous than 1D or annealing treatment at 400 °C for 50 h, where the morphology 0D nanostructures for flexible devices21. of the flake maintains during the process. AFM images (Fig. 2d) fl As a proof-of-concept demonstration, we successfully assemble show that the derived TiO2 ake remains the same shape as the = fl Ti3C2Tx (T O, OH, or F) and the derived TiO2 nanosheets into Ti3C2Tx precursor. The surface roughness of the ake also does uniform nanometer-thick films at a liquid-liquid interface, and not change much after conversion (root mean square roughness fl fabricate EC devices based on the TiO2/Ti3C2Tx heterostructures changes from 514.3 to 528.2 pm). The thickness of the ake stays fl on a exible substrate. Due to the much improved ion diffusion about 2 nm after being converted to TiO2. Typical TEM images fl and electron conductivity of assembled single- ake-layer TiO2 (Fig. 2e and Supplementary Fig. 4) and selective area electron fi lm and the 2D nature of both the MXene electrode and the TiO2 diffraction (SAED) patterns (insets of Supplementary Fig.
Details
-
File Typepdf
-
Upload Time-
-
Content LanguagesEnglish
-
Upload UserAnonymous/Not logged-in
-
File Pages11 Page
-
File Size-