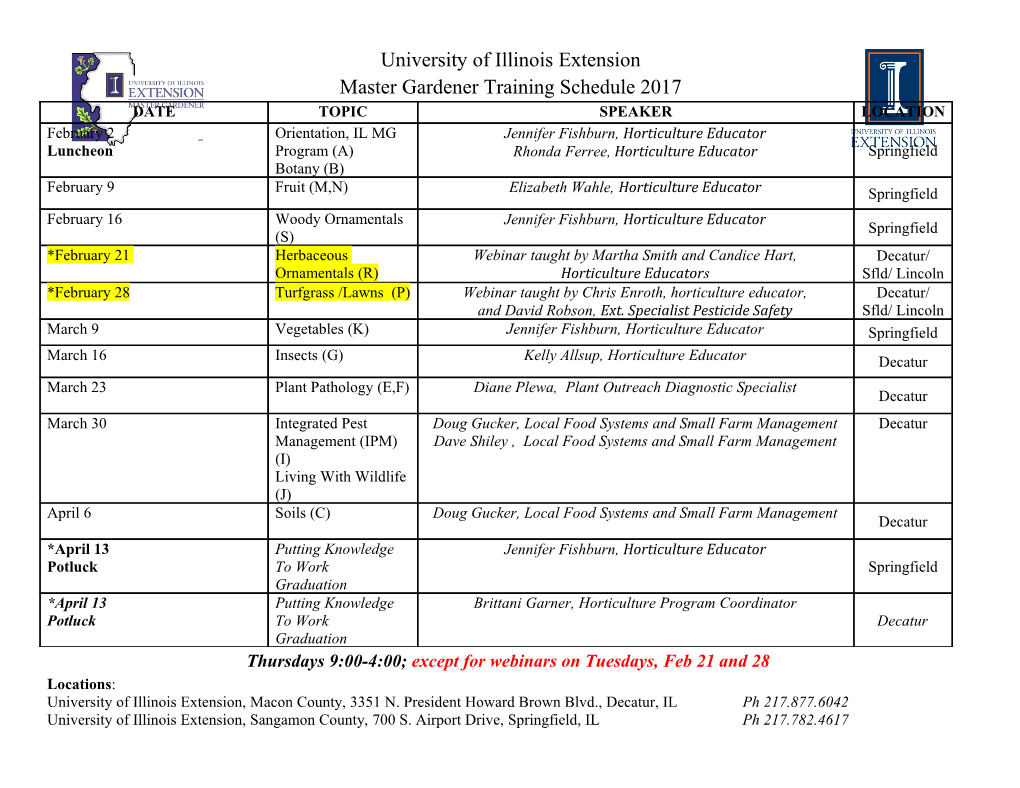
PUBLICATIONS Geophysical Research Letters RESEARCH LETTER Thunderstorms enhance tropospheric ozone 10.1002/2014GL061921 by wrapping and shedding stratospheric air Key Points: Laura L. Pan1, Cameron R. Homeyer1,2, Shawn Honomichl1, Brian A. Ridley1, Morris Weisman1, • Tropopause-reaching MCSs entrain 1 3 3 3 3 ozone-rich stratospheric air Mary C. Barth , Johnathan W. Hair , Marta A. Fenn , Carolyn Butler , Glenn S. Diskin , 3 4 4,5 4,5 into troposphere James H. Crawford , Thomas B. Ryerson , Ilana Pollack , Jeff Peischl , • Airborne lidar measurement is key to and Heidi Huntrieser6 revealing this transport mechanism • A missing transport pathway for 1National Center for Atmospheric Research, Boulder, Colorado, USA, 2Now at School of Meteorology, University of ozone budget in major global models Oklahoma, Norman, Oklahoma, USA, 3NASA Langley Research Center, Hampton, Virginia, USA, 4Chemical Sciences Division Earth System Research Laboratory, National Oceanic and Atmospheric Administration, Boulder, Colorado, USA, 5Cooperative Institute for Research in Environmental Sciences, University of Colorado Boulder, Boulder, Colorado, USA, Correspondence to: 6Institute of Atmospheric Physics, German Aerospace Center, Oberpfaffenhofen, Germany L. L. Pan, [email protected] Abstract A significant source of ozone in the troposphere is transport from the stratosphere. The Citation: stratospheric contribution has been estimated mainly using global models that attribute the transport process Pan, L. L., et al. (2014), Thunderstorms largely to the global-scale Brewer-Dobson circulation and synoptic-scale dynamics associated with upper enhance tropospheric ozone by wrap- tropospheric jet streams. We report observations from research aircraft that reveal additional transport of ping and shedding stratospheric air, Geophys. Res. Lett., 41, 7785–7790, ozone-rich stratospheric air downward into the upper troposphere by a leading-line-trailing-stratiform mesoscale doi:10.1002/2014GL061921. convective system with convection overshooting the tropopause altitude. The fine-scale transport demonstrated by these observations poses a significant challenge to global models that currently do not resolve storm-scale Received 17 SEP 2014 dynamics. Thus, the upper tropospheric ozone budget simulated by global chemistry-climate models where Accepted 5 NOV 2014 Accepted article online 7 NOV 2014 large-scale dynamics and photochemical production from lightning-produced NO are the controlling factors Published online 22 NOV 2014 may require modification. 1. Introduction Tropospheric ozone plays an important role in atmospheric chemistry and in climate forcing. Chemically, ozone controls the atmospheric oxidizing capacity via its photochemical link to OH [Brasseur et al., 1999]. It can be a major pollutant at the surface with adverse effects on human health [National Research Council, 1991]. It is a significant greenhouse gas, especially in the upper troposphere [Lacis et al., 1990; Forster and Shine, 1997], but its contribution to the radiative forcing of climate change continues to have significant uncertainty [Intergovernmental Panel on Climate Change, 2013]. The burden of tropospheric ozone is controlled by photochemical production and loss, deposition at the Earth’s surface, and net transport from the stratosphere. Changes in anthropogenic and natural emissions of ozone precursors, in circulation concomitant with climate change, and in stratospheric input all contribute to trends in tropospheric ozone. Observational and modeling studies have largely attributed transport of stratospheric ozone to the troposphere by large-scale circulation and synoptic-scale dynamical processes [Holton et al., 1995; Sprenger and Wernli, 2003; Hsu et al., 2005], such as the intrusion of stratospheric air near upper level fronts associated with the jet stream [Danielsen, 1968; Shapiro, 1980; Pan et al., 2010; Lin et al., 2012; Langford et al., 2012]. Aircraft observations made in 1985 and 1989 suggested that perturbation of the tropopause by a deep convective thunderstorm led to net ozone transport to the troposphere [Dickerson et al., 1987; Poulida et al., 1996; Stenchikov et al., 1996]. Upper tropospheric ozone enhancement from the stratosphere was also observed near thunderstorm anvils in aircraft studies described by Dye et al. [2000] and Hitchman et al. [2004], but details of the transport process remain obscure, largely because the limited sampling of in situ measurements could not provide complete information of chemical versus dynamical structures of the air mass at the cloud scale. Here we report the first unambiguous observation of thunderstorm-driven stratosphere-to-troposphere transport of ozone made during the Deep Convective Clouds and Chemistry (DC3) experiment conducted over the central U.S. in the summer of 2012. The DC3 experiment was based in Salina Kansas from mid-May to late June of 2012. PAN ET AL. ©2014. American Geophysical Union. All Rights Reserved. 7785 Geophysical Research Letters 10.1002/2014GL061921 Figure 1. (top) The vertical distribution of upper tropospheric ozone mixing ratio measured by DIAL along approximately 30 min (~400 km in distance) of the DC-8 flight leading to the location shown in Figure 2. Note that the cross section is shown in reversed time scale to ease the connection with the east to west flight track shown in Figure 2. The black color indicates the region when the DIAL ozone channel produced no data due to cloud attenuation. In situ ozone is shown by the color of the flight track. (bottom) The depolarization ratio from the DIAL 355 nm wavelength channel provides a signature of cloud/storm edge and outflow pattern in the neighborhood of the storm. The outflow structure shown in light blue is most consistent with low number density of ice particles, which complements the stretching and shedding shown in the ozone structure (Figure 1 (top)). A principal objective was to investigate the impact of deep convection on atmospheric trace gases, including ozone. Three research aircraft participated in the campaign: the National Science Foundation (NSF) and National Center for Atmospheric Research Gulfstream V (GV), the National Aeronautics and Space Administration (NASA) DC-8, and the German Aerospace Center (DLR) Falcon, each equipped with an extensive payload of meteorological and chemical sensors. The NASA Langley Differential Absorption Lidar (DIAL) was included on the DC-8 to obtain profile measurements of ozone and aerosols above and below the flight track [Hair et al., 2008]. It is the measurements from DIAL within and near thunderstorms that revealed novel evidence for the deep convective pathway of ozone transport from the stratosphere into the troposphere. 2. Ozone Observations Near Deep Convective Thunderstorms The transport event was observed on the flight of 30 May 2012 when the DC-8 aircraft passed over a large mesoscale convective system (MCS) on its return to Salina, Kansas. At the time of the overpass, the storm system had evolved into a classic leading-line trailing-stratiform (LLTS) MCS [Houze et al., 1989]. The convective overshooting behavior of this MCS has been analyzed in detail using high-resolution radar observations. The radar analysis shows that the convective elements of the storm overshot the tropopause up to 3 km. This analysis and the convective transport of tropospheric air into the stratosphere by this storm including water vapor injection into the lower stratosphere are detailed in Homeyer et al. [2014b]. Figure 1 shows that around 02 UTC of 31 May, the DC-8 was flying at 12.5 km altitude, above the tropopause located PAN ET AL. ©2014. American Geophysical Union. All Rights Reserved. 7786 Geophysical Research Letters 10.1002/2014GL061921 Figure 2. The position of the MCS and the DC-8 aircraft at 21:25 CDT 30 May 2012 (02:25 UTC 31 May). The MCS is shown using the GOES Infrared satellite cloud field (gray shading) and the Next Generation Weather Radar Weather Surveillance Radar, 1988 Doppler radar reflectivity (color shading). The DC-8 flight track is colored by the in situ ozone mixing ratio. The aircraft was at lower altitudes during most of the flight and sampling ozone in the tropospheric value range (100 ppb or lower, indicated by blue-green colors). A triangle symbol is used to mark the location of the aircraft at 01:53 UTC, corresponding to the earliest time shown in Figures 1 and 3. Thin red lines indicate the state borders. near 12 km, on a track nearly perpendicular to the leading convective line. DIAL retrieved a two-dimensional profile “curtain” of ozone along this track revealing the flow structure that has the shape of a “ram’s horn” in front of the leading edge of the storm. The edge of the storm cloud is determined from the 355 nm wavelength channel of DIAL (lower panel of Figure 1). The location and structure of the MCS and position of Figure 3. Time series of DC-8 in situ measurements for the flight time period shown in Figure 1. Note that the time axis is reversed to be consistent with Figure 1. The in situ O3 (red) and CO (blue) show anticorrelated turbulent structure during the time period of 02:08–02:15 UTC, consistent with the cloud edge indicated by the 355 nm channel (Figure 1 (bottom)). Also shown are the DC-8 flight altitude (solid black line) and the large-scale analysis of the tropopause (green dashed line). PAN ET AL. ©2014. American Geophysical Union. All Rights Reserved. 7787 Geophysical Research Letters 10.1002/2014GL061921 Figure 4. Vertical cross section of the WRF-simulated 30 May storm. The wind vectors (black arrows) show storm-relative wind, produced by subtracting the mean storm motion (14 m/s). Transport of stratospheric air is shown by the distribu- tion of a passive stratospheric tracer (blue color fill), initiated as 100% at each grid point above the tropopause (black dots). The observed wrapping of stratospheric air from the edge of the storm is reproduced by the nonzero fraction of this tracer below the tropopause and around the cloud edge (gray line). The potential temperature field (thin black lines, 2 K interval) is also included to show the dynamics of the convection.
Details
-
File Typepdf
-
Upload Time-
-
Content LanguagesEnglish
-
Upload UserAnonymous/Not logged-in
-
File Pages6 Page
-
File Size-