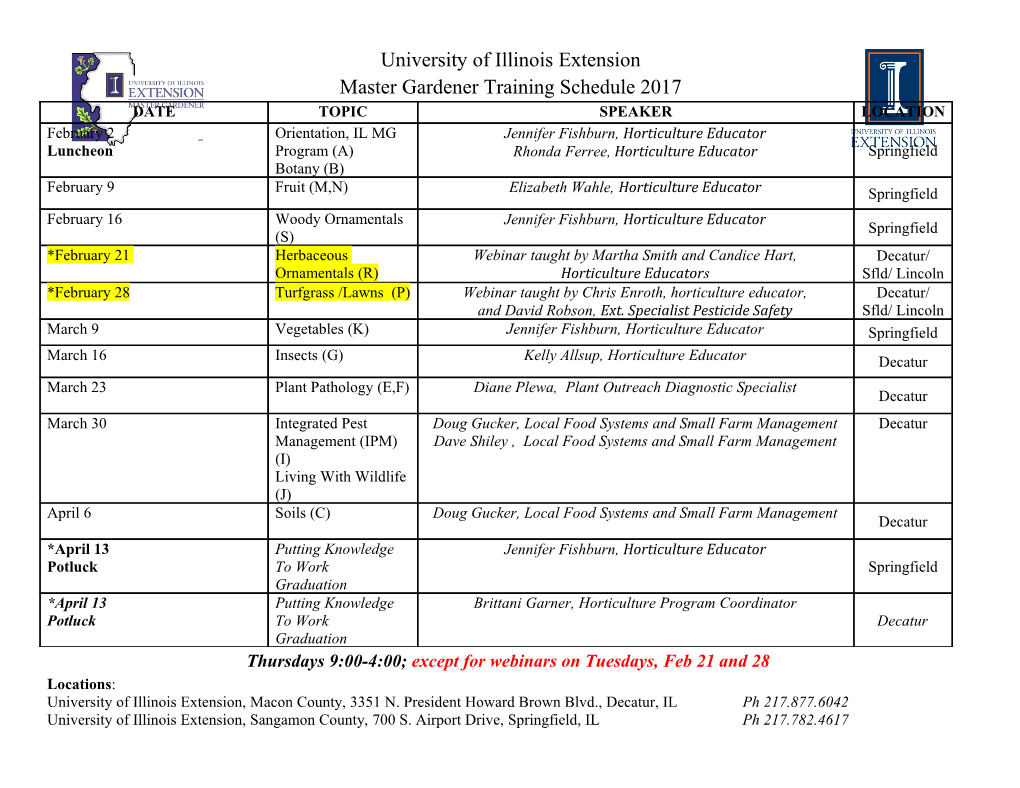
ABSTRACT Discovery of Novel 3'-Phosphoadenosine-5'-Phosphosulfate (PAPS) Reductase from Methanarcheon Methanocaldococcus jannaschii Myung Kook Cho, M.S. Mentor: Sung-Kun Kim, Ph.D. This study explores the potential assimilatory sulfate reduction pathway in methanarcheon by investigating the gene product of the open reading frame Mj0066. We expressed and purified the gene product of Mj0066 of Methanocaldococcus jannaschii to explore its substrate specificity and the reaction kinetics. Kinetic studies revealed the wild-type enzyme specifically reduced PAPS with E. coli thioredoxin supplied as the -1 -1 electron donor. The Km, Vmax, and kcat/Km value was 15.9 µM, 0.09429 µMmg min , and 5571 M-1s-1, respectively, at pH 8.0 and 30 oC. Therefore, the gene product of Mj0066 was identified as a novel 5’-phosphoadenosine-phosphosulfate reductase. Furthermore, site directed mutagenesis of individual cysteines and redox titration were performed in order to investigate the catalytic mechanism. The combined studies show Cys337 plays a role in substrate binding while Cys19 and Cys22 are involved in electron transfer. Taken together, PAPS-reductase activity advocates the presence of a PAPS-utilizing assimilatory sulfate reduction pathway M. jannaschii. Discovery of Novel 3'-Phosphoadenosine-5'-Phosphosulfate (PAPS) Reductase from Methanarcheon Methanocaldococcus jannaschii by Myung Kook Cho, B.A. A Thesis Department of Chemistry and Biochemistry Patrick J. Farmer, Ph.D., Chairperson Submitted to the Graduate Faculty of Baylor University in Partial Fulfillment of the Requirements for the Degree of Master of Science Approved by the Thesis Committee Sung-Kun Kim, Ph.D., Chairperson Bryan F. Shaw, Ph.D. Kenneth T. Park, Ph.D. Accepted by the Graduate School August 2013 J. Larry Lyon, Ph.D., Dean Page bearing signatures is kept on file in the Graduate School. Copyright © 2013 by Myung Kook Cho All rights reserved TABLE OF CONTENTS LIST OF FIGURES ··············································································· v LIST OF TABLES ··············································································· vi LIST OF ABBREVIATIONS ··································································· vii ACKNOWLEDGEMENTS ····································································· viii CHAPTER ONE ···················································································· 1 Introduction ························································································ 1 CHAPTER TWO ··················································································· 7 Materials and Methods ··········································································· 7 Bioinformatic ·················································································· 7 Cloning of Mj0066 ············································································· 7 Construction of Mj0066 Mutant Plasmids ·················································· 8 Expression of the Recombinant Wild- Type and Mutant Proteins ······················· 8 Purification of the Recombinant Wild- Type and Mutant Proteins ···················· 10 Enzymatic Assays ············································································· 11 Dependence of PAPR Activity/Inhibition Based on PAPS Concentration ············ 11 Ultraviolet-Visible Scanning of Purified Proteins ········································ 12 Mass Spectrometry ··········································································· 12 Nonreducing SDS-PAGE ···································································· 13 Redox Potential of MjPAPR Wild Type and Mutants by mBBr Protein Titration ····13 CHAPTER THREE ··············································································· 15 Results ···························································································· 15 ORF Mj0066 Encodes Methanocaldococcus jannaschii PAPS Reductase ··········· 15 Role of Cysteine Residue in the Catalytic Mechanism ··································· 19 Role of Trx in the Catalytic Mechanism ··················································· 25 CHAPTER FOUR ················································································· 31 Discussion ························································································ 31 Determination of PAPS Reductase in M. jannaschii ····································· 31 Active Site of PAPS Reductase in M. jannaschii ·········································· 31 A Distinct Enzyme-Thiosulfonate Intermediate and A Novel CXXC Redox Motif ··· 36 Sulfonucleotide Reduction Mechanism for MjPAPR ····································· 38 Ecological Implication ······································································· 41 Evolutionary Insight on Sulfate Reduction Pathway in Methanogens······································································41 CHAPTER FIVE ·················································································· 44 Conclusion ······················································································· 44 APPENDIX ························································································ 46 Supplementary Figures ········································································ 47 REFERENCES ···················································································· 57 iv LIST OF FIGURES Figure 1. Sulfate assimilation pathway ·························································· 2 Figure 2. Methanogenesis pathway ······························································· 4 Figure 3. Structure of substrates and product of methanogenesis ···························· 5 Figure 4. SDS-PAGE of purified rMj00066 and mutants ···································· 16 Figure 5. Multiple sequence alignment of Mj0066 with E. coli and S. cervasiae PAPS-Reductase ··································································· 17 Figure 6. UV/vis spectrum analysis of MjPAPR ·············································· 18 Figure 7. Deconvolution of MjPAPR mass spectrum with ESI-orbitrap ·················· 21 Figure 8. Deconvolution of mutant C19A mass spectrum with ESI-orbitrap ··············22 Figure 9. Deconvolution of mutant C22A mass spectrum with ESI-orbitrap ············· 23 Figure 10. Deconvolution of mutant C337A mass spectrum with ESI-orbitrap············24 Figure 11. Dependence of PAPS activity and substrate inhibition based on concentration of PAPS and Thioredoxin ······················································· 26 Figure 12. Michaelis-Menton curve of MjPAPR using PAPS and Trx as independent substrates ····································································26 Figure 13. Reciprocal plot analysis of MjPAPR ·············································· 27 Figure 14. Non-reducing SDS-PAGE of MjPAPR and Trx C35A ··························28 Figure 15. Redox Titration Curve of MjPAPR wildtype ····································· 28 Figure 16. Redox Titration Curve of MjPAPR variant C337A ····························· 29 Figure 17. Redox Titration Curve of MjPAPR variant C19A ······························· 29 Figure 18. Redox Titration Curve of MjPAPR variant C22A ······························· 30 Figure 19. Crystal structure of EcPAPR ······················································· 32 Figure 20. Crystal structure of ScPAPR ························································ 33 Figure 21. Proposed PAPS reduction mechanism for MjPAPR ···························· 40 v LIST OF TABLES Table 1. Sequences of oligonucleotide primers used in site directed mutagenesis PCR amplification ························································ 9 Table 2. Kinetic parameters of MjPAPR ······················································· 18 Table 3. PAPS activation of wild type and variants of MjPAPR ··························· 20 Table 4. Comparison of kinetic parameters of MjAPR and MjPAPR ······················ 43 vi LIST OF ABBREVIATIONS APR — Adenosine 5’-phosphosulfate reductase APS — Adeonsine 5’-phosphosulfate Asn — Asparagine ATP — Adenosine triphosphate CoB — Coenzyme B CoM — Coenzyme M Cys — Cysteine DNA — Deoxyribonucleic acid E. coli — Escherichia coli EcPAPR — Escherichia coli PAPR EDTA — Ethylenediaminetetraacetic acid ESI-MS — Electrospray ionization mass spectrometry Grx — Glutaredoxin HEPES — 4-(2-hydroxyethyl)-1-piperazineethanesulfonic acid H4MPT — Tetrahydromethanopterin IPTG — Isopropyl-beta-D-thioglactopyranoside LB — Luria broth LPLC — Low pressure liquid chromatography MES — 2-(N-morpholino)ethanesulfonic acid MFR – Methanofuran Mj — Methanocaldococcus jannaschii MjAPR — Methanocaldococcus jannaschii APR MjPAPR — Methanocaldococcus jannaschii PAPR NADPH — Nicotinamide adenine dinucleotide phosphate NCBI — National Center for Biotechnology Information ORF — Open reading frame PAGE — Polyacrylamide gel electrophoresis PAPR — 3’-phosphoadenosine-5’-phosphosulfate reductase PAPS — 3’-phosphoadenosine-5’-phosphosulfate PCR — Polyacrylamide gel electrophoresis PDB — Protein data bank RMSD — Root mean square S. cerevisiae — Saccharomyces cerevisiae ScPAPR — Saccharomyces cerevisiae PAPR SDS — Sodium dodecyl sulfate Thr — Threonine Trx — Thioredoxin Tyr — Tyrosine UV — Ultraviolet VIS — Visible vii ACKNOWLEDGEMENTS I would like to thank Dr. Sung-Kun Kim and Jong-sun Lee for the project and being my mentors. I would also like to thank my parents for helping
Details
-
File Typepdf
-
Upload Time-
-
Content LanguagesEnglish
-
Upload UserAnonymous/Not logged-in
-
File Pages68 Page
-
File Size-