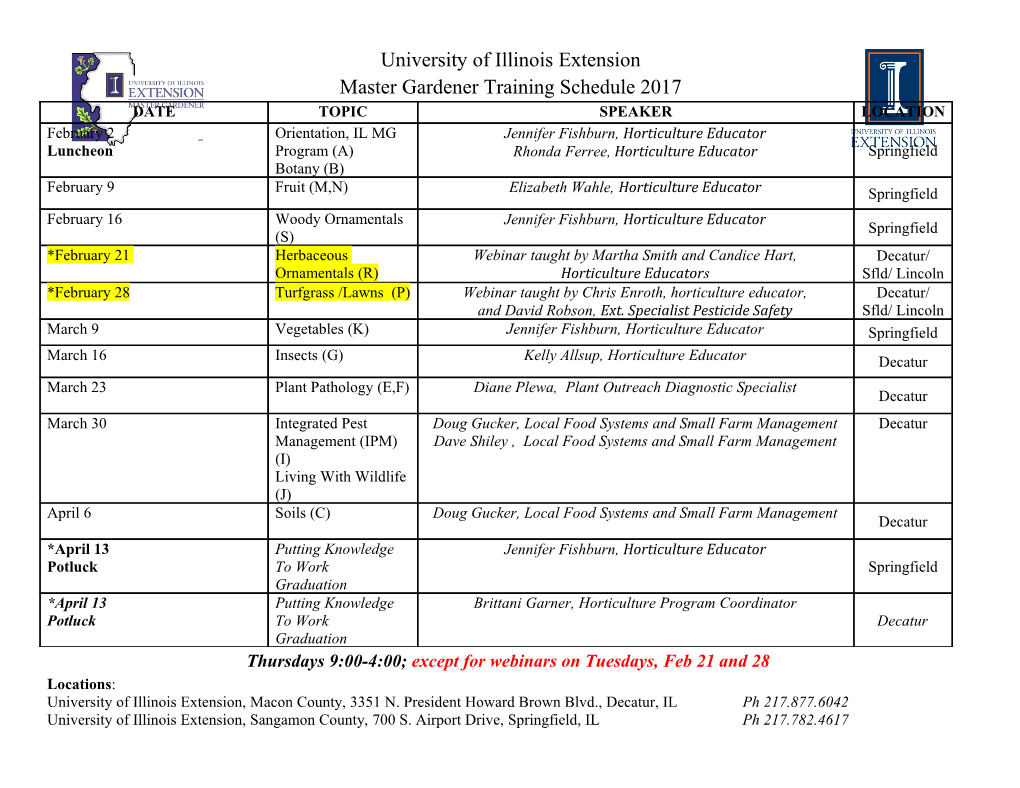
Structure, catalytic mechanism, and membrane interaction of the mTOR activator Rheb by Mohammad Taghi Mazhab Jafari A thesis submitted in conformity with the requirements for the degree of Doctor of Philosophy Department of Medical Biophysics University of Toronto © Copyright by Mohammad Taghi Mazhab Jafari 2014 Structure, catalytic mechanism, and membrane interaction of the mTOR activator Rheb Mohammad Taghi Mazhab Jafari Doctor of Philosophy Department of Medical Biophysics University of Toronto 2014 Abstract The activator of mammalian target of rapamycin complex 1 (mTORC1), Ras homolog enriched in brain (Rheb), is a membrane-associated protein belonging to the Ras subfamily of small GTPases. Rheb’s slow GTPase activity is stimulated by the GTPase activating protein (GAP) domain of tuberous sclerosis complex 1 and 2 (TSC1/2). Rheb hyperactivation, through its overexpression or loss of TSC1/2 GAP function, results in hyperactivated mTORC1 signaling culminating in tumourigenesis. The molecular details of Rheb GTP hydrolysis and the effect of membrane association on Rheb structure, dynamics and its GTPase function are currently not fully understood. The studies presented in this thesis focus on two key determinants of Rheb function i) the mechanism of Rheb GTP hydrolysis and ii) the structural and functional consequence of Rheb-bilayer membrane interaction. Through studies of fluorescent nucleotides, we revealed that the conserved G2-box residue Tyr35 auto-inhibits GTP hydrolysis in Rheb. We demonstrated that a non-canonical catalytic residue, Asp65, in the switch II region of Rheb, contributed more to the GTP hydrolysis rate than Gln64, which corresponds to the canonical Ras Gln61. This non-canonical auto-inhibited mechanism of GTP hydrolysis was required for optimal mTORC1 regulation. These structural insights were then used to guide the design of novel gain- and loss-of function mutants by substitutions of the ultra-conserved G3-box Gly63 of Rheb. Finally, using solution ii NMR spectroscopy, we monitored the Rheb GTPase cycle and characterized its nucleotide-dependent membrane orientations on nanodisc-based phospholipid bilayers. Rheb was shown to sample two orientations in which its C-terminal helix was semi-perpendicular or semi-parallel with respect to the bilayer plane. The semi-parallel orientation, where switch II residues critical for mTORC1 communication are accessible, was favored in the GTP bound conformation, suggesting that membrane- tethering modulates Rheb function. These structural insights into the catalytic machinery of Rheb and its membrane interface suggest new approaches to modulate these key determinants of Rheb function through small molecules towards development of therapeutic avenues for Rheb-mediated pathogenesis such as tuberous sclerosis or cancer. iii Acknowledgments First and foremost, I would like to thank my supervisor Dr. Mitsu Ikura for his guidance, support, and understanding. I could have not accomplished any portion of this research without his supervision. His passion for science was a continuous source of inspiration for me over the years. I learned key aspects of successful research from him, including critical thinking. I am grateful to my supervisory committee members; Dr. Lewis Kay and Dr. Vuk Stambolic. Their expert inputs were critical to the success of this thesis. Thank you for your advise, supervision and encouragement, which kept me on the right path. I am also thankful to my collaborators, Vanessa De Palma and Jason Ho from Dr. Stambolic lab for the cell biology experiments. I had the honor of working with and learning from many excellent current and former members of Ikura’s lab including; Dr. Christopher Marshall, Dr. Peter Stathopulos, Dr. Genevieve Seabrook, Dr. Noboru Ishiyama, Le Zheng, Dr. Feng Wang, Carol Liu, and Dr. Fernando Amador. I would like to specially thank Chris, who was involved in all aspects of my projects and gave valuable inputs and suggestions. Finally, I would like to thank my family; my parents Mohammad Mazhab-Jafari and Ziba Fadavi and my brother Hamed Mazhab-Jafari for all their sacrifice that provided me with the opportunity to carry out this research. Words cannot express my grateful feelings for you. iv Table of Contents Acknowledgements……………………………………………………………………………………….iv Table of Contents ………………………………………………………………………………………...v List of Tables …………………………………………………………………………………………….x List of Figures …………………………………………………………………………………………...xi List of Appendices ……………………………………………………………………………………...xiv List of Abbreviations …………………………………………………………………………………...xv Chapter 1: Introduction and Thesis Overview ………………………………………………………...1 1.1 General introduction to small GTPase proteins……………………………………………………….2 1.2 Introduction to Rheb………………………………………………………………..............................3 1.3 Biophysical and biochemical properties of Rheb…………………………………..............................6 1.4 Pathogenesis of Rheb………………………………………………………………………………….9 1.5 Thesis Overview and Rationale……………………………………………………………………...11 1.6 Attributions…………………………………………………………………………………………..12 1.7 References……………………………………………………………………………………………12 Chapter 2: Fluorescent-tagged Nucleotides Alter the Native GTPase Cycle …………………….…17 2.1 Abstract………………………………………………………………………………………………18 2.2 Introduction………………………………………………………………………..............................19 2.3 Results………………………………………………………………………………………………..20 2.3.1 The effect of mant on the intrinsic rate of nucleotide hydrolysis………………………….20 v 2.3.2 The effect of mant on the rate of GAP-catalyzed nucleotide hydrolysis…………………..23 2.3.3 The effect of mant on the GEF-mediated nucleotide exchange…………………………....25 2.4 Discussion……………………………………………………………………………………………28 2.4.1 Intrinsic hydrolysis of GTP and mantGTP………………………………………………...28 2.4.2 GAP-catalyzed GTP hydrolysis by HRas and Rheb…………………………………….…30 2.4.3 GEF-accelerated nucleotide exchange of HRas and RhoA………………………………..31 2.5 Experimental Procedures…………………………………………………………………………….32 2.5.1 Protein preparation…………………………………………………………………………32 2.5.2 NMR-based GTPase, GAP and GEF assays……………………………………………….33 2.5.3 Fluorescence-based GTPase assay…………………………………………………………34 2.6 References……………………………………………………………………………………………34 Chapter 3: Mechanism of GTP hydrolysis by Rheb ……………………………………………..…...36 3.1 Abstract…………………………………………………………………………………………..…..37 3.2 Introduction………………………………………………………………………………………..…38 3.3 Results………………………………………………………………………………………………..39 3.3.1 Rheb Tyr35 inhibits intrinsic GTPase activity……………………………………………..39 3.3.2 Structural basis for the Tyr35 auto-inhibitory function……………………………………40 3.3.3 Identification of a catalytic residue for GTP hydrolysis…………………………………...42 3.3.4 Involvement of Rheb’s Asp65 and Tyr35 in TSC2GAP-mediated GTP hydrolysis…......47 3.3.5 Thermodynamic basis for the Tyr35 auto-inhibitory function…………………………….49 vi 3.3.6 Regulation of mTORC1 by growth factors involves the non-canonical catalytic and autoinhibitory mechanisms…………………………………………………………….………...54 3.4 Discussion……………………………………………………………………………………….…...57 3.5 Experimental Procedures……………………………………………………………………….……60 3.5.1 Protein preparation………………………………………………………………….……...60 3.5.2 Crystallization and data collection………………………………………………….……...61 3.5.3 Structure determination and refinement…………………………………………….……...61 3.5.4 NMR-based GTPase assays…………………………………………………………….…62 3.5.5 Thermodynamic measurements……………………………………………………….…..63 3.5.6 Cell-based phosphorylation assay……………………………………………………..…...63 3.5.7 Nucleotide binding in vivo…………………………………………………………………64 3.6 References……………………………………………………………………………………………65 Chapter 4: Structure-guided design of novel active and inactive Rheb mutants using single site modifications ……………………………………………………………………………………………68 4.1 Abstract……………………………………………………………………………………………....69 4.2 Introduction…………………………………………………………………………………………..70 4.3 Results and Discussion……………………………………………………………………………....72 4.4 Experimental Procedures…………………………………………………………………………….78 4.4.1 Protein Preparation…………………………………………………………………………78 4.4.2 NMR-based Real-time GTPase assay……………………………………………………...78 4.4.3 Crystallization and Data Collection………………………………………………………..79 vii 4.4.4 Structure Determination and Refinement………………………………………………….79 4.4.5 Cell-based Phosphorylation Assays………………………………………………………..80 4.5 References……………………………………………………………………………………………82 Chapter 5: Structural and Functional consequence of Rheb-membrane interaction ……………...84 5.1 Abstract………………………………………………………………………………………………85 5.2 Introduction…………………………………………………………………………………………..86 5.3 Results and Discussion………………………………………………………………………………88 5.4 Experimental Procedures……………………………………………………………………….……99 5.4.1 Protein preparations………………………………………………………………….…….99 5.4.2 Preparation of Rheb-nanodisc complex…………………………………………………..100 5.4.3 NMR- measurements……………………………………………………………………..101 5.4.4 Real-time NMR-based GTPase assay………………………………………………….…103 5.4.5 Molecular docking simulation……………………………………………………………103 5.5 References……………………………………………………………………………………….….106 Chapter 6: Conclusions and Future directions ……………...………………………………………108 6.1 Conclusions…………………………………………………………………………………………109 6.2 Future Directions…………………………………………………………………………………...110 6.2.1 Engineering GTPase probes by structure-guided mutations of G3-box glycine………....110 6.2.2 Drugging Rheb……………………………………………………………………………111 6.2.3 Probing membrane-dependent regulation of KRas-effector interaction………………….111 viii 6.3 Closing Remarks……………………………………………………………………………………112 6.4 References…………………………………………………………………………………………..114 Appendix A……………………………………………………………………………………………..116
Details
-
File Typepdf
-
Upload Time-
-
Content LanguagesEnglish
-
Upload UserAnonymous/Not logged-in
-
File Pages136 Page
-
File Size-