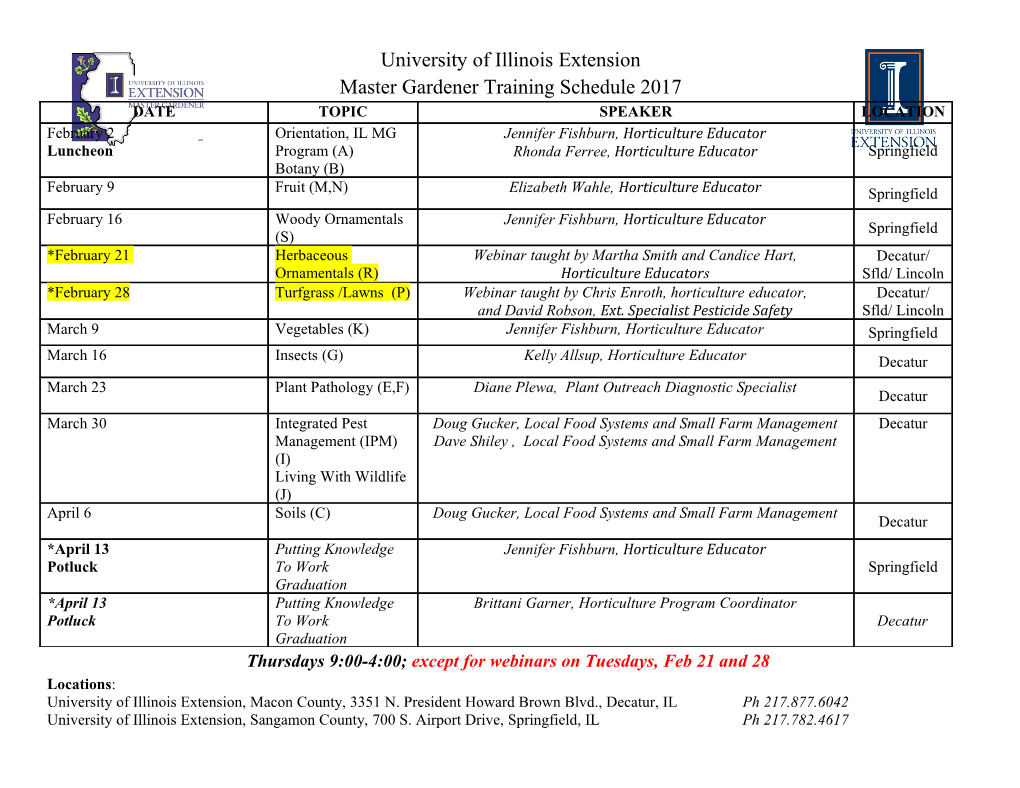
AE 451 Aeronautical Engineering Design I Estimation of Critical Performance Parameters Prof. Dr. Serkan Özgen Dept. Aerospace Engineering November 2017 Airfoil selection • The airfoil effects the cruise speed, takeoff and landing distances, stall speed, handling qualities and overall aerodynamic efficiency during all phases of flight. • The airfoil may be separated into: – Thickness distribution, influences profile drag, – Zero-thickness camber line, influences lift and drag due to lift. • Upper surface of an airfoil or wing produces roughly 2/3 of total lift. • Zero-lift angle of attack is roughly equal to the percent camber of the airfoil (in deg). 2 Design lift coefficient • This is the lift coefficient at which the airfoil has the best L/D. • The airplane should be designed such that it flies the design mission at or near the design lift coefficient to maximize the aerodynamic efficiency. 3 Airfoil geometry 4 Stall • Some airfoils show a gradual reduction in lift during stall, while others show a violent loss of lift with a rapid change in pitching moment. 5 Stall • Thick airfoils (round leading edge, t/c>14%) stall starting from the trailing edge. At around α=10o, the boundary-layer begins to separate starting at the trailing edge and moving forward as the angle of attack is further increased. The loss of lift is gradual, pitching moment does not change significantly. • Moderately thick airfoils (6%<t/c<14%) stall from the leading edge. Flow separates over the nose at a very low angle of attack, but immediately reattaches, so the effect is initially small. At some higher α, the flow does not reattach and the airfoil stalls almost immediately. Lift and pitching moment vary violently. 6 Stall • Thin airfoils (t/c<6%) stall from the leading edge and the flow reattaches immediately. As α is increased, the bubble contnues to stretch towards the trailing edge as the angle of attack is increased. At α where the bubble stretches all the way to the trailing edge, cl,max is reached. Beyond that α, the flow is separated over the entire airfoil, so stall occurs. The loss of lift is smooth, but large changes in pitching moment are observed. • Twisting the wing such that the tip airfoils have a reduced angle of attck compared to the root (washout) can cause the wing to stall at the root first. 7 Stall • Different airfoil sections may be used at the root and the tip, with a tip airfoil that stalls at a higher angle of attack. This produces good flow over the ailerons for roll control (aileron authority) at an angle of attack where the root has stalled. • Stall characteristics for thinner airfoils may be improved with leading edge devices like slots, leading edge flaps, etc. • Wing stall is directly related to airfoil stall only for high aspect ratio, unswept wings. For low aspect ratio, swept wings, 3-D effects dominate stall characteristics and airfoil stall characteristics can be ignored. • Horizontal tail or canard size is directly related to the magnitude of the wing pitching moment to be balanced. 8 Thickness ratio • Airfoil thickness ratio has a direct effect on drag, maximum lift, stall characteristics and structural weight. • A wing with a fairly high AR, moderate sweep, large nose radius provides a higher stall angle and a higher CL,max. • For a wing with low AR, swept wings, a sharper leading edge provides greater CL,max due to the formation of vortices behind the leading edge. • Wing structural weight ~1/ 푡/푐 • halving the thickness ratio increases the empty weight of the airplane by 6%. 9 Thickness ratio 10 Thickness ratio 11 Thickness ratio 12 Thickness ratio • For initial selection of the thickness ratio, historical trends can be used. Supercritical airfoils can be chosen 10% thicker. 13 Thickness ratio • In subsonic airplanes, the root airfoil can be 20-60% thicker than the tip airfoil without effecting the drag due to fuselage effects. This thicker root should not extend beyond 30% of span. • This results in a structural weight reduction as well as more volume for fuel and landing gear. • Each airfoil is designed for a certain Reynolds number. Use of an airfoil at greatly different Reynolds numbers produce section characteristics much different than expected. This is especially true for laminar flow airfoils. 14 Wing geometry • The reference or trapezoidal wing is the basic geometry to begin the layout. • The leading edge sweep is important for supersonic flight. In order to reduce drag, it is important to sweep the wing leading edge behind the Mach cone. 15 Wing geometry • The quarter chord sweep is related to the subsonic flight since the lift produced by a wing is proportional to the component of the freestream velocity vector perpendicular to the quarter chord line. 16 Wing geometry • For a complete trapezoidal wing, the aerodynamic center is at the quarter chord point of the mean aerodynamic chord. • In supersonic flow, the aerodynamic center moves approximately back to 40% of the mean aerodynamic chord. 17 Aspect ratio • For finite aspect ratio wing, tip vortices lower the pressure difference between the upper and lower surfaces. This reduces the lift near the wingtip. • The tip vortices reduce the effective angle of attack of the wing, more so at the wingtips. • A high aspect ratio wing has wingtips further apart compared to an equal area wing with low AR. Therefore, the amount of wing effected by the wingtip is less for a high aspect ratio wing and the strength of the wingtip vortex is reduced. loss of lift and induced drag is less for high aspect ratio wing. 퐿/퐷)푚푎푥~ 퐴푅 푊푤푛푔~ 퐴푅 18 Lift to drag ratio • L/D is a measure of overall aerodynamic efficiency. – Subsonic speeds: L/D=L/D(wing span,wetted area) – Supersonic speeds: L/D=L/D(wing span, wetted area, Mach) • Drag components at subsonic speeds: – Induced drag or drag due to lift is a function of the wing span – Parasite drag or zero lift drag is a function of total surface area exposed to air 2 L/D is a function of “wetted aspect ratio”=b /Swet 19 Wetted aspect ratio 20 Aspect ratio • Due to reduced effective angle of attack of the wingtips, a low AR wing will stall at a higher angle of attack compared to a high aspect ratio wing. • This is why tails have low AR compared to wings. • This ensures adequate control even when the wing stalls. 21 Aspect ratio 22 Wing sweep • Wing sweep is used primarily to reduce the adverse effects of transonic and supersonic flow. • The leading edge sweep must be such that it is behind the Mach cone. • Theoretically, the shock wave formation on a swept wing is determined by the air velocity in a direction perpendicular to the leading edge of the wing. • In the transonic flow regime, wing sweep is determined by the requirement for a high critical Mach number, Mcrit. This requires subsonic airflow over the airfoil measured perpendicular to the leading edge, thus a swept wing. 23 Wing sweep 24 Wing sweep • The exact wing sweep selection depends on the selected airfoil, thickness ratio, taper ratio, etc. 25 Wing sweep • Wing sweep improves lateral stability (roll). A swept wing has a natural dihedral effect 10o sweep ≈ 1o dihedral. • It may be necessary to use zero or negative dihedral on a swept wing in order to avoid a stiff airplane. • The wing sweep and aspect ratio together have a strong effect on the pitch-up characteristics. • Pitch-up is a highly undesirable tendency of some aircraft near the stall angle to suddenly and uncontrollably increase the angle of attack. 26 Wing sweep 27 Taper ratio • An elliptical wing will produce the lowest induced drag but is difficult and more costly to produce. • A tapered wing is almost equally efficient in terms of induced drag. 28 Taper ratio 29 Taper ratio • There are two competing considerations: ― Smaller the taper ratio, lighter the wing structure. If λ is less, more lift will be produced at the wingroot center of pressure moves towards the wing root and the moment arm from the wingroot to the center of pressure decreases and the bending moment at the root decreasing the need for heavier structure. ― Wings with low λ show undesirable stall characteristics. Separation at the root has two advantages: Turbulent flow trailing downstream from the root causes buffeting as it flows over the tail, giving a strong stall warning to the pilot. The wingtips have attached flow so the ailerons will be more efficient. 30 Taper ratio • Low sweep wing: typically have taper ratios around 0.4-0.5. • High sweep wings: have taper ratios around 0.2-0.3. • A swept wing will direct the air outward towards the wingtips. • This loads up the wingtips creating more lift there compared to an equivalent unswept wing. • In order to restore the elliptic lift distribution, it is necessary to reduce the taper ratio. 31 Taper ratio 32 Twist • Wing twist is used to prevent tip stall and to revise the lift distribution to approximate an elliptical one. • Typically, wings are twisted between 0o-5o. • Geometric twist is the actual change in airfoil angle of incidence measured with respect to the root airfoil. • A wing with a tip airfoil at a negative angle compared to the root airfoil has «washout». For such a wing, the root will stall before the tip, which improves aileron control at high α and tends to reduce wing rock. • If a wing has linear twist, the twist angle changes in proportion to the distance from the wingroot. 33 Twist • Aerodynamic twist = 훼퐿=0,푟표표푡 − 훼퐿=0,푡푝 • Optimizing the lift distribution by twisting the wing will be valid only for one geometric angle of attack.
Details
-
File Typepdf
-
Upload Time-
-
Content LanguagesEnglish
-
Upload UserAnonymous/Not logged-in
-
File Pages69 Page
-
File Size-