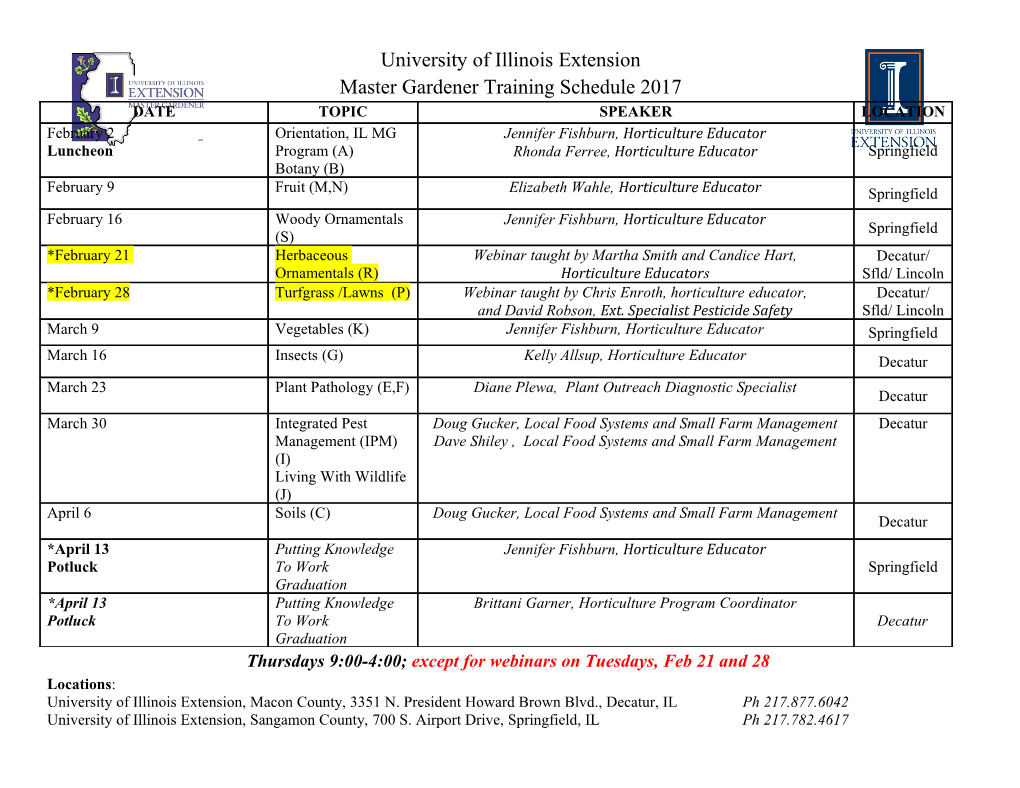
contents Principles of Biology 23 ATP and Cellular Work ATP provides the energy that powers cells. Magnetic resonance images of three different areas in the rat brain show blood flow and the biochemical measurements of ATP, pH, and glucose, which are all measures of energy use and production in brain tissue. The image is color-coded to show spatial differences in the concentration of these energy-related variables in brain tissue. © 1997 Nature Publishing Group Hoehn-Berlage, M., et al. Inhibition of nonselective cation channels reduces focal ischemic injury of rat brain. Journal of Cerebral Blood Flow and Metabolism 17, 534–542 (1997) doi: 10.1097/00004647-199705000-00007. Used with permission. Topics Covered in this Module Using Energy Resources For Work ATP-Driven Work Major Objectives of this Module Describe the role of ATP in energy-coupling reactions. Explain how ATP hydrolysis performs cellular work. Recognize chemical reactions that require ATP hydrolysis. page 116 of 989 4 pages left in this module contents Principles of Biology 23 ATP and Cellular Work Energy is a fundamental necessity for all of life's processes. Without energy, flagella cannot move, DNA cannot be unwound or separated for replication or gene expression, cells cannot divide, plants cannot grow and animals cannot reproduce. Energy is vital, but where does it come from? Plants and photosynthetic microbes capture light energy and convert it into chemical energy for their own use. Organisms that cannot produce their own food, such as fungi and animals, feed upon this captured energy. However, the chemical energy produced by photosynthesizers needs to be converted into a usable form. Otherwise, it is like going to a grocery store with a bag of gold — until the gold is converted to the local currency, it is useless. For most organisms, that local currency is the molecule ATP. Using Energy Resources For Work Many molecules can be used to store energy, but only one is called "the energy currency of the cell." What are the characteristics of a molecule that stores energy? How is this energy used by the cell to do work? Understanding the chemistry behind these questions will lead us to a more thorough understanding of how cells are able to conduct the business of living. The molecule ATP functions as an energy carrier. Adenosine triphosphate (ATP) is composed of the nitrogenous base adenine, the sugar ribose, and three phosphate groups (Figure 1). Figure 1: The structure of ATP. ATP is composed of the nitrogenous base adenine, a ribose sugar, and a chain of three phosphate groups. The unstable phosphoanhydride bonds permit transfer of phosphate groups to other molecules. © 2014 Nature Education All rights reserved. Energy is stored in the phosphoanhydride bonds between the three phosphate groups in ATP. This energy is released by ATP hydrolysis, the chemical reaction in which ATP reacts with water to yield adenosine 2- diphosphate (ADP) and an inorganic phosphate ion, denoted HOPO3 or Pi (Figure 2). For every mole of ATP hydrolyzed, 7.3 kilocalories of energy are released: ATP + H2O → ADP + Pi ΔG = -7.3 kcal/mol The standard Gibbs free energy (ΔG) is the portion of free energy that can be used by a system to perform work when temperature and pressure are uniform. It is a very handy number that can be used to predict whether a reaction will occur spontaneously within a cell. Exergonic reactions, such as ATP hydrolysis, release energy and have negative ΔG values. Conversely, endergonic reactions, which do not begin spontaneously and require an input of energy, have positive ΔG values. Figure 2: The hydrolysis of ATP. A water molecule hydrolyzes ATP into ADP and inorganic phosphate (Pi 2- or HOPO3 ) by breaking the bond between the two terminal phosphate groups in ATP. The reaction also releases energy and a proton. Energy is released because the bond energy in the phosphoanhydride bond is greater than the energy in the bonds of the products. © 2013 Nature Education All rights reserved. A common misconception is that the phosphate bonds of ATP are high-energy bonds. However, there is nothing special about the bonds themselves. In reality, the energy release comes from the reaction between water and ATP, which results in the hydrolysis of the phosphate bonds. The reactants, water and ATP, have higher energy than the products, ADP and Pi, providing the energy commonly associated with ATP. But why are the products of ATP hydrolysis at lower energy than the reactants? ATP has three phosphate groups, all of which have negative charges. The close proximity of these charges destabilizes ATP because of the high degree of electrostatic repulsion. On the other hand, ADP has only two phosphate groups, which results in less electrostatic repulsion, lower energy, and therefore a more stable molecule. Furthermore, the other product of ATP hydrolysis also exhibits energetically favorable properties. Not only is Pi isolated from other negative charges, but it can be stabilized by interactions with water molecules in the cell (Figure 3). Taken together, Pi and ADP are at a much lower energy level than ATP. The hydrolysis of ATP releases this difference in energy. Figure 3: Ball and stick models of ATP, ADP and inorganic phosphate (Pi). ATP (a) is less chemically stable than ADP (b) because ADP has less electrostatic repulsion between negatively charged phosphate groups. Inorganic phosphate (c) has four oxygen atoms with partial negative charges that can be stabilized by water molecules in biological systems. These qualities make ADP and Pi much more stable than ATP. Red, blue, gray, white and orange spheres represent oxygen, nitrogen, carbon, hydrogen and phosphorus atoms, respectively. © 2013 Nature Education All rights reserved. ATP powers energetically unfavorable reactions in the cell. How does the hydrolysis of ATP lead to the cell carrying out useful reactions? The release of energy only explains half the story. On its own, the hydrolysis of ATP would simply result in organisms overheating because the dissipation of energy would excite nearby molecules, resulting in heat (thermal energy). For energy to be useful in a cell, it needs to be linked to other processes. Energy coupling is the transfer of energy from one chemical reaction to another. By coupling the release of energy that occurs during ATP hydrolysis with changes in protein conformation, the breaking and forming of chemical bonds, and other reactions that require energy, the cell can perform nearly all of the tasks it needs to function. How is ATP used during energy-coupling reactions? As an example, consider the phosphorylation of the sugar glucose into glucose-6-phosphate (Figure 4). This is the first step in glycolysis, one of the most fundamental processes for generating ATP in all organisms. The direct addition of an inorganic phosphate group to glucose will not occur spontaneously because it involves a positive free energy change (ΔG > 0) — it is endergonic. To overcome this problem, cells couple glucose phosphorylation to ATP hydrolysis through a two-step process catalyzed by the enzyme hexokinase. First, ATP is hydrolyzed into ADP and an inorganic phosphate group. The hydrolysis of ATP is exergonic, involving a negative free energy change (ΔG < 0). The inorganic phosphate group released from the first reaction is then transferred to glucose, forming glucose-6-phosphate. This initial investment of energy is needed to completely break down glucose in later steps of glycolysis, which release more net energy. Figure 4: The phosphorylation of glucose is coupled with ATP hydrolysis. In this reaction, the addition of an inorganic phosphate group (Pi) to glucose to form glucose-6-phosphate — the first step of glycolysis — is energetically unfavorable (∆G = +3.3 kcal/mol). The hydrolysis of ATP into ADP and Pi is energetically favorable (-7.3 kcal/mol). Coupling the two reactions allows the energy released during ATP hydrolysis to be used to drive the endergonic reaction. © 2014 Nature Education All rights reserved. Figure Detail The ΔG of the direct phosphorylation of glucose is +3.3 kcal/mol, and the ΔG of ATP hydrolysis is -7.3 kcal/mol. By coupling these reactions, the ∆G values of each reaction are summed, giving an overall ∆G of -4.0 kcal/mol (+3.3 kcal/mol + (-7.3 kcal/mol) = -4.0 kcal/mol). Because the free energy change of the overall reaction is negative, the overall reaction is energetically favorable and will occur spontaneously. In this way, ATP hydrolysis can be used to provide the free energy needed to drive reactions that would otherwise be energetically unfavorable (Figure 5). Figure 5: Spontaneous reactions. Chemical reactions will occur spontaneously if the free energy of the reaction is negative. © 2013 Nature Education All rights reserved. Transcript The cycling of ATP. The exergonic hydrolysis of ATP is coupled to many, but not all, endergonic reactions in the cell. It fuels the movement of muscles, the movement of molecules into and out of cells, chemical reactions, and more. Humans use approximately 50 kg of ATP each day. However, not even Olympic sprinters can store 50 kg of ATP in their muscles; at most, they store approximately one minute's worth of ATP. Obviously, ATP must be regenerated continuously. The addition of an inorganic phosphate to ADP regenerates ATP. This reaction, the phosphorylation of ADP, is endergonic and requires +7.3 kcal/mol of energy. (Note that the opposite reaction, the hydrolysis of ATP, releases the same amount of energy.) ADP + Pi → ATP + H2O ΔG = +7.3 kcal/mol For most life forms, the energy required to regenerate ATP comes from respiration of the chemical energy initially stored during photosynthesis; this stored energy is used by either the organism that did the photosynthesis itself or an organism that consumed the products of that photosynthesis.
Details
-
File Typepdf
-
Upload Time-
-
Content LanguagesEnglish
-
Upload UserAnonymous/Not logged-in
-
File Pages15 Page
-
File Size-