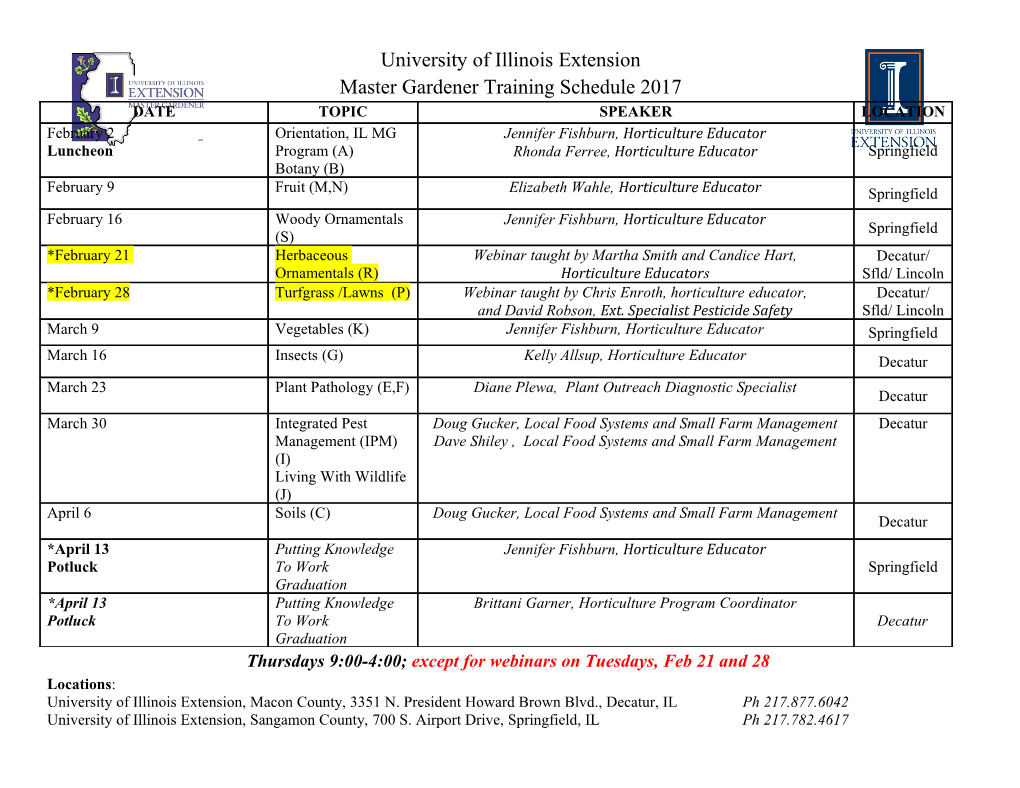
Article Global upper-atmospheric heating on Jupiter by the polar aurorae https://doi.org/10.1038/s41586-021-03706-w J. O’Donoghue1,2 ✉, L. Moore3, T. Bhakyapaibul3, H. Melin4, T. Stallard4, J. E. P. Connerney2,5 & C. Tao6 Received: 25 February 2021 Accepted: 8 June 2021 Jupiter’s upper atmosphere is considerably hotter than expected from the amount of Published online: 4 August 2021 sunlight that it receives1–3. Processes that couple the magnetosphere to the atmosphere Open access give rise to intense auroral emissions and enormous deposition of energy in the Check for updates magnetic polar regions, so it has been presumed that redistribution of this energy could heat the rest of the planet4–6. Instead, most thermospheric global circulation models demonstrate that auroral energy is trapped at high latitudes by the strong winds on this rapidly rotating planet3,5,7–10. Consequently, other possible heat sources have continued to be studied, such as heating by gravity waves and acoustic waves emanating from the lower atmosphere2,11–13. Each mechanism would imprint a unique signature on the global Jovian temperature gradients, thus revealing the dominant heat source, but a lack of planet-wide, high-resolution data has meant that these gradients have not been determined. Here we report infrared spectroscopy of Jupiter with a spatial resolution of 2 degrees in longitude and latitude, extending from pole to equator. We fnd that temperatures decrease steadily from the auroral polar regions to the equator. Furthermore, during a period of enhanced activity possibly driven by a solar wind compression, a high-temperature planetary-scale structure was observed that may be propagating from the aurora. These observations indicate that Jupiter’s upper atmosphere is predominantly heated by the redistribution of auroral energy. Jupiter was observed with the 10-m Keck II telescope for five hours on data (see Methods). Using a magnetic field model, we have overlaid both 14 April 2016 and 25 January 2017 using NIRSPEC (Near-InfraRed oval-shaped lines on the polar regions of Jupiter in both Figs. 2 and 3, Spectrometer14), with the spectral slit aligned north–south along the with each representing the footprint of magnetic field lines that trace axis of planetary rotation (Fig. 1a). Spectral images were acquired as from the planet out to a particular distance in Jupiter’s equatorial 18 Jupiter rotated, as shown in Fig. 1b, c, in which rotational–vibrational plane . The main (auroral) oval traces on average to 30RJ in Jupiter’s + (ro-vibrational) emission lines of the H3 ion can be seen extending equatorial plane (RJ is Jupiter’s equatorial radius of 71,492 km at the from pole to equator. These ions are a major constituent of Jupiter’s 1-bar pressure level). The satellite footprints of Io and Amalthea are ionosphere and mainly reside in the altitude range 600–1,000 km fiducial markers, mapping out from the planet to 5.9RJ and 2.54RJ in above the 1-bar pressure surface15. The intensity ratio of two or more the equatorial plane, respectively. + H3 lines can be used to derive the column-averaged parameters of that Temperatures generally decrease from 1,000 K to 600 K between 16 + ion: temperature, number density and radiance . As H3 is assumed auroral latitudes and the equator, as shown in Figs. 2 and 3. Densities of + to be in quasi-local thermodynamic equilibrium with Jupiter’s upper H3 , which are enhanced by aurorally driven charged-particle precipi- atmosphere16, its derived temperature is representative of the region. tation19,20, cut off sharply near the main oval on both dates, indicating + Details of the H3 fitting process and global mapping of parameters are that the direct influence of the aurora ends within several degrees of provided in the Methods and in Extended Data Figs. 1, 2. the main oval. At the same time, equatorward of the auroral regions, + Global maps of upper-atmospheric temperature have been pro- H3 temperatures do not sharply fall with latitude. In the absence of duced previously17, but the spatial resolution was such that about any known sub-auroral electric current systems (as are common on two pixels covered 45–90° latitude in each hemisphere, making it Earth21) provided through magnetosphere–ionosphere coupling that difficult to assess how the auroral region was connected to the rest cause planetary-scale ion–neutral collisions, we interpret the observed of the planet. In those maps, equatorial temperatures were similar temperature gradients as strong evidence that the auroral upper atmos- to auroral values, a finding that would indicate that a heat source is phere is migrating away from the auroral region to lower latitudes active at low latitudes. In Figs. 2 and 3, we show near-global maps of and adjacent longitudes, transporting its heat signature along with + Jovian column-averaged H3 temperature, density and radiance, which it. This must then be enabled principally by equatorward-propagating are the product of several thousand individual fits to the spectral meridional winds. 1Department of Solar System Science, JAXA Institute of Space and Astronautical Science, Sagamihara, Japan. 2NASA Goddard Space Flight Center, Greenbelt, MD, USA. 3Center for Space Physics, Boston University, Boston, MA, USA. 4Department of Physics and Astronomy, University of Leicester, Leicester, UK. 5Space Research Corporation, Annapolis, MD, USA. 6National Institute of Information and Communications Technology (NICT), Tokyo, Japan. ✉e-mail: [email protected] 54 | Nature | Vol 596 | 5 August 2021 a b c –1 60 )] –2 –1 sr –1 40 –3 m μ –2 m –4 [Spectral radiance 20 10 (W –5 log Planetocentric latitude (º N) 0 3.42 3.43 3.44 3.45 3.46 3.95 3.96 3.97 3.98 3.99 4.00 Wavelength (μm) + Fig. 1 | Example set-up showing the acquisition of Jovian spectra on 14 April sunlight from hydrocarbons and hazes. Well-defined vertical lines are H3 2016. a, Slit-viewing camera image filtered between 2.134 μm and 4.228 μm ro-vibrational emission lines: they are most intense in the polar regions. The + wavelength. Guide images such as this are taken every 9 s and indicate the slit’s R(3,0) and Q(1,0) H3 lines at 3.41277 μm and 3.9529 μm are the focus of this position on the sky relative to Jupiter. In this image, the Great Red Spot (bottom study, as their consistently high signal-to-noise (SNR) at all latitudes allows us + left) and satellite Ganymede (top left) can be seen. b, c, Spectral images of to map upper-atmospheric energy balance globally. The SNR of H3 is high at Jupiter showing spectral radiance as a function of wavelength and Jupiter owing to the convenient presence of a deep methane absorption band, planetocentric latitude. Most emissions seen in c are from the reflection of particularly in b (ref. 27). 14 April 2016 25 January 2017 a 90 d 90 75 75 1,000 60 60 ) 45 45 900 30 30 Amalthea 15 15 800 + Io + 0 T(H3 ) Main oval 0 T(H3 ) –15 –15 700 –30 –30 temperature (K) + 3 –45 –45 600 H –60 –60 + Planetocentric latitude (° –75 –75 500 –90 –90 360 330 300 270 240 210 180 150 120 90 60 30 0 360 330 300 270 240 210 180 150 120 90 60 30 0 b 90 e 90 75 75 ) 16 60 60 10 ) 45 45 –2 30 30 Amalthea 15 Io 15 + N(H +) 0 N(H3 ) Main oval 0 3 –15 –15 –30 –30 –45 –45 column density (m + 3 –60 –60 H Planetocentric latitude (° –75 –75 1015 –90 –90 360 330 300 270 240 210 180 150 120 90 60 30 0 360 330 300 270 240 210 180 150 120 90 60 30 0 c 90 f 90 75 75 60 60 ) –1 –3 45 45 10 sr 30 30 –2 Amalthea m 15 Io 15 + E(H +) 0 E(H3 ) Main oval 0 3 10–4 –15 –15 –30 –30 –45 –45 10–5 radiance (W + 3 –60 –60 H Planetocentric latitude (°) –75 –75 –90 –90 360 330 300 270 240 210 180 150 120 90 60 30 0 360 330 300 270 240 210 180 150 120 90 60 30 0 System III central meridian longitude (°) System III central meridian longitude (°) + Fig. 2 | Equirectangular projections of Jupiter’s H3 column-integrated text). White indicates regions with no data coverage (or where no results met temperature, density and radiance. Projections are shown as a function of the uncertainty criteria). Median (and maximum) uncertainty percentiles central meridian longitude (Jovian system III) and planetocentric latitude. for 14 April 2016 are: temperature 2.2% (5%), density 9.4% (15%) and radiance Temperature (T) and radiance (E) panels have uncertainties below 5%, while 2.2% (5%). Median (and maximum) uncertainties for 25 January 2017 are: column densities (N) are limited to 20%. Long black-and-white dashed lines temperature 1.6% (5%), density 5.8% (15%) and radiance 1.8% (5%). The Methods show Jupiter’s main auroral oval, short black-and-white dashed lines describes the mapping process, and Extended Data Tables 1–3 show the bin correspond to the magnetic footprint of Io, and the single thick black line sizes that were used in each parameter map. corresponds to the magnetic footprint of Amalthea (described in the main Nature | Vol 596 | 5 August 2021 | 55 Article a + 14 April 2016 25 Jan. 2017 H3 temperature (K) 1,000 900 800 700 600 240° 120° 500 180° 180° b 1,150 1,100 14 April 2016 1,050 25 January 2017 1,000 950 900 850 800 750 Median temperature (K) 700 650 600 550 90 75 60 45 30 15 0 –15 –30 –45 –60 –75 –90 Planetocentric latitude (°) + Fig.
Details
-
File Typepdf
-
Upload Time-
-
Content LanguagesEnglish
-
Upload UserAnonymous/Not logged-in
-
File Pages14 Page
-
File Size-