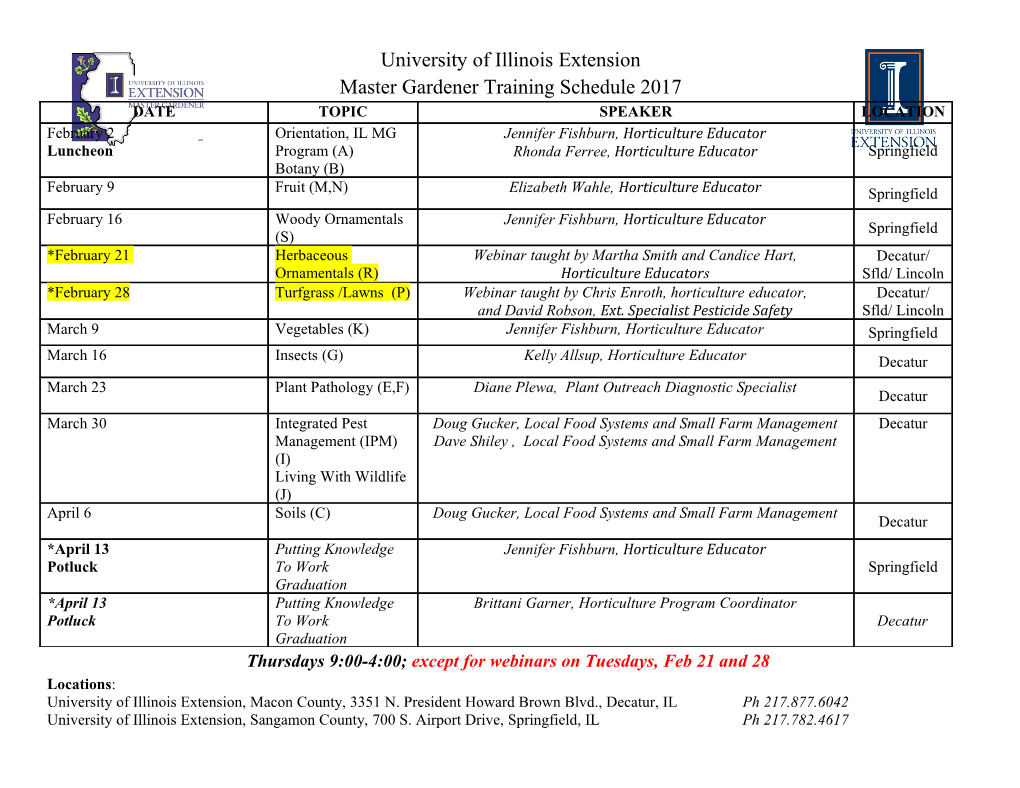
Towing Asteroids with Gravity Tractors Enhanced by Tethers and Solar Sails Haijun Shen∗ and Carlos M. Roithmayr† Material collected from an asteroid’s surface can be used to increase gravitational attraction between the asteroid and a Gravity Tractor (GT); the spacecraft therefore operates more effectively and is referred to as an Enhanced Gravity Tractor (EGT). The use of tethers and solar sails to further improve effectiveness and simplify operations is investigated. By employing a tether, the asteroidal material can be placed close to the as- teroid while the spacecraft is stationed farther away, resulting in a better safety margin and improved thruster efficiency. A solar sail on a spacecraft can naturally provide radial offset and inter-spacecraft separation re- quired for multiple EGTs. I. Introduction Collisions between objects that make up our solar system are commonplace, as evidenced by numerous craters on planets, moons, asteroids, and comets. In fact, it has been theorized that most of the boulders and regolith on asteroids such as 21 Lutetia, 433 Eros, etc., are formed by such collisions.1–3 The planet Earth is not immune from the danger. Even though our atmosphere protects the Earth by incinerating smaller objects (sub 10-m range) before they reach the surface, larger objects can survive the atmospheric transit and cause catastrophic damage. The extinction of the dinosaurs and many other species is attributed to a huge asteroid, more than 10 km in diameter, that hit the Yucatan Peninsula in Mexico sixty-five million years ago. In 1908, Tunguska, Siberia was devastated by a small object roughly 50 meters across. Although it exploded in the air before it hit the ground, it nevertheless flattened 60 million trees. It would have erased St. Petersburg had it arrived four hours and fifty-two minutes later.4 More recently, the 300-m asteroid 1989 FC missed the Earth by merely six hours on March 23rd, 1989.5 An impact in the ocean from an asteroid of this size can cause a tsunami with 300 times more energy than the one caused by the 2004 Sumatra-Andaman earthquake, which killed 200,000 people.6 On February 15th, 2013, the Chelyabinsk meteor survived atmospheric transit and exploded in an air burst at a height of roughly 29.7 km, creating many small meteorites that reached the ground. The airburst released a total kinetic energy that was 20–30 times the amount produced by the atomic bomb detonated at Hiroshima. Even though most of the energy was absorbed by the atmosphere, it caused infrastructure damage and seriously injured as many as 1,500 people.7 The threat is real, and the best conceivable mitigation consists of detecting and deflecting potentially hazardous ob- jects. Various ways of deflecting an asteroid have been proposed. High-energy schemes utilizing kinetic impactors8–10 and nuclear or non-nuclearblasts11 are capable of achieving large deflection in a short period of time, which is effective if a threat is detected with a short warning time. These schemes are not suitable for rubble-pile asteroids held together by weak gravity and cohesion. For smaller asteroids with a long warning time, a few low-energy schemes have been proposed. In Ref. [12], Bombardelli and Pelaez suggest blasting an asteroid surface with ion beams, which transfers momentum from the ion particles to the asteroid. Mechanical attachment of a propulsion system to an asteroid is problematic. First, it may be difficult to obtain firm attachment if the asteroid is a rubble pile or composed of low-strength material. Second, an asteroid’s rotational motion interferes with application of thrust in the best direction for deflection. These problems are solved with a concept proposed by Lu and Love in Ref. [13]; it involves no physical contact and is known as the Gravity Tractor (GT). A spacecraft is placed so that it leads the asteroid in its heliocentric trajectory. Two thrusters impart momentum to the spacecraft, which tugs the asteroid through mutual gravitational attraction. The thrusters are canted in order to prevent impingement of the plume streams on the asteroid surface. However, the portion of thrust that contributes to effective asteroid deflection is proportional to the cosine of the cant angle; wasted thrust is proportional to the sine ∗Analytical Mechanics Associates, Inc., 21 Enterprise Parkway, Suite 300, Hampton, VA 23666, USA +1 757 865 0000, [email protected] †NASA Langley Research Center, Vehicle Analysis Branch, MS 451, 1 North Dryden Street, Hampton, VA, 23681, USA +1 757 864 6778, [email protected] 1 of 12 American Institute of Aeronautics and Astronautics of the cant angle. The disadvantage of thruster cant is addressed by McInnes in Ref. [14] by placing the spacecraft in a halo orbit in front of the asteroid. A properly sized halo orbit makes it possible to prevent plume impingement without canting the thrusters. This idea is extended by Wie in Ref. [15] to include multiple spacecraft in a halo orbit to expedite deflection. Another innovation introduced in Ref. [15] is the addition of a solar sail to the gravity tractor. The sail is oriented so as to provide a component of Solar Radiation Pressure (SRP) force in the direction of the asteroid’s motion and impart momentum to the asteroid-spacecraft system. A gravity tractor can be made more effective by adding mass to the spacecraft because doing so increases the gravitational attraction between the asteroid and the spacecraft. Collecting boulders and regolith from the asteroid surface is an attractive way to add mass to the spacecraft.16, 17 In-situ utilization of native asteroidal material reduces the launch cost and trip time associated with sending the same amount of mass from Earth. Compared to regular GT operations without the additional mass, the Enhanced Gravity Tractor (EGT) is more effective, and thus, requires less time to achieve the same amount of deflection. Reference [17] shows that the deflection times can be reduced by a factor of 10 to 50 or more with EGT compared to GT, depending on the propulsion system’s capability and the mass collected. In this paper we explore the use of a tether and a solar sail to make an EGT more effective and provide operational simplification. A container of asteroidal material is attached to a tether and placed close to the asteroid to increase gravitational attraction, while the spacecraft is placed farther away from the asteroid for a better margin of safety. In the case of a single EGT placed in front of the asteroid, as in Ref. [13], the increased distance afforded by a tether permits a smaller thruster cant angle. Operationally, the halo orbit employed in Ref. [14] requires tight orbit control. Instead of using a halo orbit to provide lateral displacement of the spacecraft, we employ a solar sail. In the case of a single spacecraft placed in the asteroid’s heliocentric orbit plane and slightly farther away from the sun than the asteroid, as in Ref. [15], the cant angle of the towing thrusters can be eliminated. The solar sail can be oriented so that it provides only the lateral displacement, or so that it also provides some of the towing force. Two additional spacecraft with solar sails can be placed to either side of the asteroid’s heliocentric orbit plane; in this case, their solar sails can be oriented so as to maintain the out-of-plane positions. Figure 1: In-track EGT with a tether II. In-Track EGT with Tether One of the major disadvantages of the GT proposed by Lu and Love13 is the thrust cant angle, which leads to an expenditure of propellant that does not directly contribute to deflecting the asteroid. The GT can be made more effective by moving the spacecraft closer to the asteroid to increase the gravitational attraction, but this increases the cant angle and, furthermore, reduces safety margin for the spacecraft. The cant angle can be made smaller by moving the spacecraft further away from the asteroid, but doing so reduces the gravitational attraction, and thus diminishes the effectiveness of the GT. If the spacecraft and the collected boulder mass are collocated, then the EGT is affected by the 2 of 12 American Institute of Aeronautics and Astronautics 1 0.8 θ 0.6 cos 0.4 0.2 3 2 30 25 1 20 15 0 10 L / R α (deg) Figure 2: Thruster efficiency as a function of plume cone half-angle and tether length same disadvantage. The dilemma can be avoided by furnishing the EGT with a tether. That is, the material collected from the asteroid is attached to a tether and placed where the EGT would have been without the tether, or even closer to the asteroid. With the asteroidal material on the tether, the spacecraft can be moved further away from the asteroid, as shown in Fig. 1, reducing the thruster cant angle and improving the safety margin for the spacecraft. The reduction in the cant angle varies, depending on the length of the tether and the distance from the asteroid to the asteroidal material. The asteroidal material obtained from the surface could be a single monolithic boulder, multiple boulders, or a collection of regolith; in this paper we will use the terms “asteroidal material” and “boulder” interchangeably. In Fig. 1, xˆ is a unit vector that has the same direction as the velocity of the asteroid’s mass center in heliocentric orbit; henceforth, this is referred to as the in-track direction. The asteroid is modeled as a sphere of radius R with uniform mass distribution. α denotes the half-angle of the plume cone, r is the distance from the collected material to the mass center of the asteroid, and L is the length of the tether.
Details
-
File Typepdf
-
Upload Time-
-
Content LanguagesEnglish
-
Upload UserAnonymous/Not logged-in
-
File Pages12 Page
-
File Size-