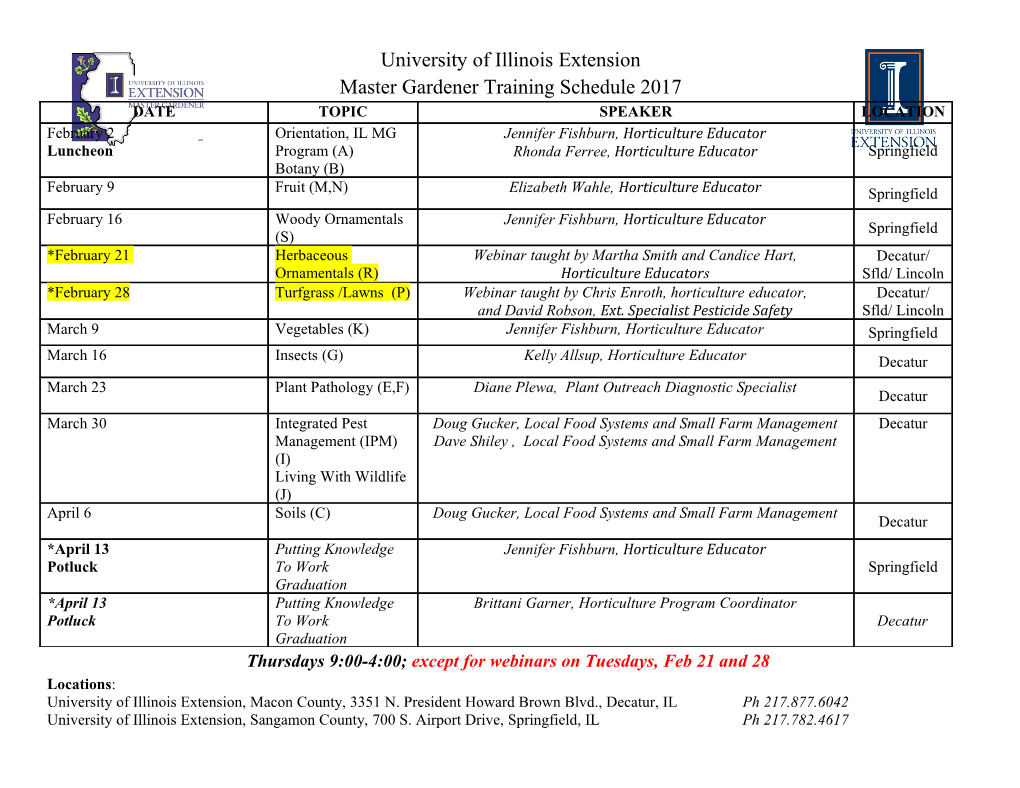
This article appeared in a journal published by Elsevier. The attached copy is furnished to the author for internal non-commercial research and education use, including for instruction at the authors institution and sharing with colleagues. Other uses, including reproduction and distribution, or selling or licensing copies, or posting to personal, institutional or third party websites are prohibited. In most cases authors are permitted to post their version of the article (e.g. in Word or Tex form) to their personal website or institutional repository. Authors requiring further information regarding Elsevier’s archiving and manuscript policies are encouraged to visit: http://www.elsevier.com/copyright Author's personal copy Journal of Experimental Marine Biology and Ecology 409 (2011) 1–12 Contents lists available at SciVerse ScienceDirect Journal of Experimental Marine Biology and Ecology journal homepage: www.elsevier.com/locate/jembe The reef coral two compartment proton flux model: A new approach relating tissue-level physiological processes to gross corallum morphology Paul L. Jokiel ⁎ Hawaii Institute of Marine Biology, University of Hawaii, P. O. Box 1346, Kaneohe, HI 96744, USA article info abstract Article history: A comparison of the equations for photosynthesis and calcification in reef corals suggests that the two processes Received 26 May 2011 compete for available inorganic carbon; yet reef corals exhibit simultaneous high rates of photosynthesis and Received in revised form 4 October 2011 calcification during daylight hours. Also, the extreme metabolic activity observed in corals at high irradiance Accepted 4 October 2011 requires a large net efflux of protons at sites of rapid calcification and respiration. Corals have resolved these Available online 26 October 2011 problems through development of morphologies that separate the zone of rapid calcification (ZC) from the zone of rapid photosynthesis (ZP), with the fixed-carbon energy supply from the ZP being rapidly translocated Keywords: Boundary layer to the ZC. Translocation of photosynthate from the ZP serves as a means of transporting protons to the ZC, Calcification where they are readily dissipated into the water column. Observations on the spatial relationship of the ZC Coral and ZP, analysis of net proton flux, incorporation of photosynthate translocation coupled with an understanding Ocean acidification of the importance of boundary layers (BL) leads to a unified hypothesis that describes the processes involved in Photosynthesis coral metabolism. The proposed model is based on the observation that reef corals have evolved a wide range of morphologies, but all of them place the ZC between the ZP and the external seawater. This spatial arrangement places the BL in contact with the ZC in order to facilitate efflux of protons out of the corallum. Placement of the ZC − between the ZP and the BL maximizes recycling of the metabolic products O2 and HCO3 . Furthermore, this arrangement maximizes the photosynthetic efficiency of zooxanthellae by producing a canopy structure with the skeletal material in the ZC serving to absorb ultraviolet radiation (UVR) while scattering photosynthetically active radiation (PAR) in a manner that maximizes absorption by the zooxanthellae. The ZP is isolated from the water column by the ZC and the BL. Therefore ZP must exchange metabolic materials with the ZC and with the water column through the ZC and its overlying BL. The resulting configuration is highly efficient and responsive to irradiance direction, irradiance intensity, water motion and coral polyp morphology. The skeletons of corals are thereby passively modified in response to physical factors such as light and water motion regime. The model presents a unified theory of coral metabolism and provides explanations for many paradoxes of coral biology, including plasticity of the diverse growth forms and an explanation for coral skeletal growth response to ocean acidification. © 2011 Elsevier B.V. All rights reserved. Contents 1. Introduction ............................................................... 2 2. Background information ......................................................... 3 2.1. The role of carbonic anhydrase (CA) in coral metabolism ....................................... 3 2.2. Relationships between coral photosynthesis and calcification ..................................... 4 2.3. Spatial relationships ........................................................ 4 2.3.1. Spatial patterns of zooxanthellae distribution ........................................ 4 2.3.2. Spatial patterns of skeletal growth ............................................. 4 2.3.3. Spatial hydrodynamic regimes ............................................... 4 2.3.4. Primary and secondary calcification spatial patterns ..................................... 5 2.4. Mechanisms controlling material flux................................................ 6 2.5. Balancing proton flux....................................................... 6 ⁎ Tel.: +1 808 236 7440; fax: +1 808 236 7443. E-mail address: [email protected]. 0022-0981/$ – see front matter © 2011 Elsevier B.V. All rights reserved. doi:10.1016/j.jembe.2011.10.008 Author's personal copy 2 P.L. Jokiel / Journal of Experimental Marine Biology and Ecology 409 (2011) 1–12 3. Synthesis of existing metabolic data into a model .............................................. 6 3.1. Primary calcification, photosynthesis and translocation of photosynthate . ............................ 6 3.2. Secondary calcification and photosynthesis ............................................. 7 3.3. Calcification in light and darkness .................................................. 8 4. Application of model at the corallum level of organization .......................................... 8 4.1. Corallum morphologies....................................................... 8 4.2. Plasticity of corallum growth form in relation to water motion and irradiance . ............................ 8 4.3. Other responses of the corallum to hydrodynamic regime ......................................10 4.4. Irradiance vs. hydrodynamics as forcing functions in computer simulations . ............................10 4.5. Evolutionary considerations. ....................................................10 5. Conclusions ...............................................................11 Acknowledgments ...............................................................11 References ..................................................................11 1. Introduction and an inner gastrodermis separated by a jelly-like substance called mesoglea (Fig. 1). Likewise, the basal body wall consists of the calicoder- A recent paper (Jokiel, 2011) describes the “proton flux hypothesis” mis and a gastrodermis separated by mesoglia. The coelenteron connects which states that the decreasing calcification rate observed in corals the polyps of a colony and opens to the external sea water through their under increasing conditions of ocean acidification can be attributed to mouths. The surface body wall epidermis contains nematocysts. The sym- higher [H+] in the seawater. Increasing [H+]inthewatercolumnwillde- biotic zooxanthellae are located mainly within the cells of the gastroder- crease the strength of the diffusion gradient which in turn will slow the mis in the surface body wall. efflux of H+ through the boundary layer and slow the rate of calcification. The zooxanthellae are photosynthetic and capable of providing all of The initial presentation of the hypothesis regarded the corallum as a the energy needed by the coral symbiosis (Muscatine et al., 1984). The “black box” and only considered the inputs and outputs through the basal wall consists of two cell layers: the cell layer adjacent to the skeleton boundary layer (BL). The purpose of the present paper is to expand is called the calicodermis. A layer of gastrodermis lies between the these observations in relation to processes within the “black box” and to calicodermis and the coelenteron and separated from the calicodermis synthesize existing data into a corallum-based model. Previous studies by mesoglea. have focused on the physiology of coral cells and tissues, without The discovery that calcification in reef corals is enhanced by light consideration of the immense complexity and diversity of corallum (Kawaguti and Sakumoto, 1948) led to development of models that morphologies. The proposed unified model is intended to address the attempted to explain the mechanisms involved. Over the past processes of photosynthesis, calcification, and translocation of 50 years a number of observations, hypotheses and reef coral calcifica- photosynthate in relation to morphology, irradiance field, hydrodynamic tion models have been presented (reviewed by Allemand et al., 2011; environment and BL conditions of the intact corallum. Cohen and Holcomb, 2009; Gattuso et al., 1999). Goreau (1959) Reef corals are coelenterates formed by a surface body wall and a basal proposed that calcification is accelerated in light due to removal of body wall that enclose a space called the coelenteron. Terminology used CO2 from calcification sites by photosynthetic zooxanthellae. This here follows that of Galloway et al. (2007). The surface body wall in model requires placement of the zooxanthellae at or near the calcifica- contact with sea water consists of two tissue layers — an outer epidermis tion sites, but they actually are located far from the site of calcification. McConnaughey Allemand Goreau (1959)
Details
-
File Typepdf
-
Upload Time-
-
Content LanguagesEnglish
-
Upload UserAnonymous/Not logged-in
-
File Pages13 Page
-
File Size-