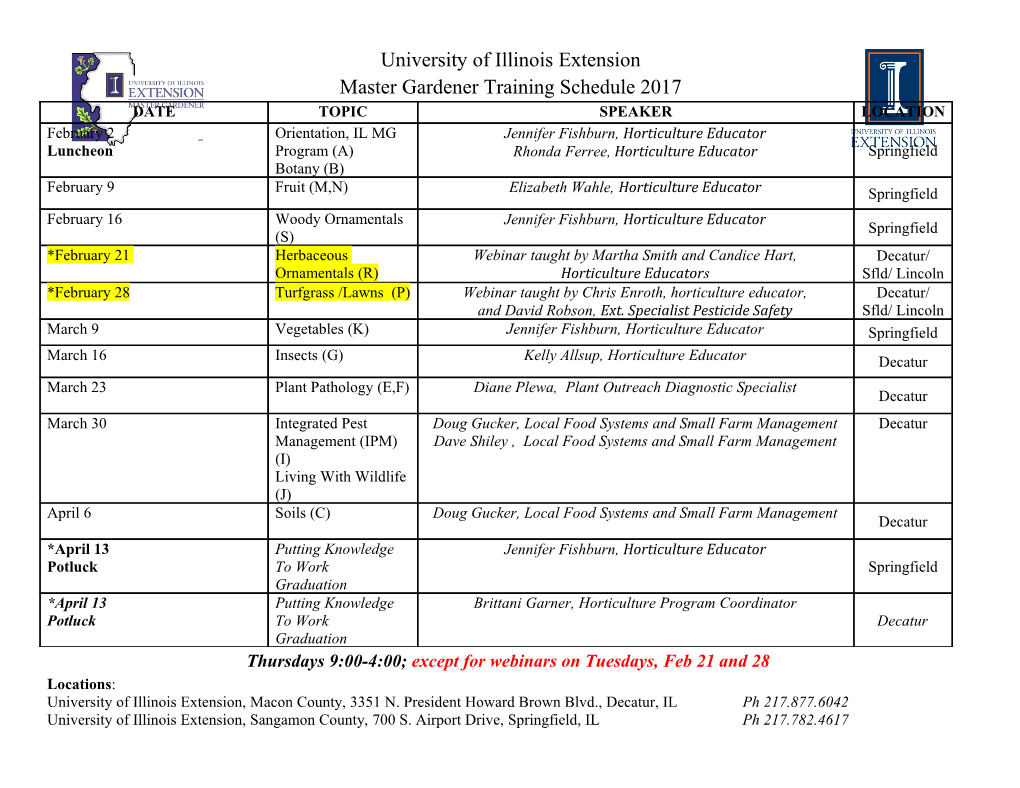
Experimental Validation of Swarm Array Results using Mars Cube One (MarCO) A/B Downlink Signals Victor Vilnrotter, Marco Quadrelli, Richard Hodges, Saptarshi Bandyopadhyay, Susan Finley, Daniel Kahan Jet Propulsion Laboratory, California Institute of Technology 4800 Oak Grove Dr., Pasadena, CA 91109 (818) 354-2745 [email protected] Abstract— In this paper, we investigate the feasibility of Mars, transmitting coherently phased signals back to calibrating an orbiting phased array composed of Earth. Swarms of low-cost SmallSats can deliver a CubeSat class spacecraft together with a larger reference comparable or even greater mission capability than (or “chief”) spacecraft, comprising an overall Swarm large monolithic spacecraft, but with significantly Array. Considered as an analog to the Deep Space enhanced flexibility (adaptability, scalability, Network (DSN) Uplink Arraying problem [1, 2], a evolvability, and maintainability) and robustness spaceborne Swarm Array can potentially deliver comparable or even greater operational performance (reliability, survivability, and fault-tolerance). than large monolithic spacecraft (such as increased data- rates for telecommunications, or greater baselines for improved spatial resolution), thus increasing future mission capability but with significantly enhanced flexibility, evolvability and robustness. TABLE OF CONTENTS 1. INTRODUCTION ....................................................... 1 2. DATA ACQUISITION AT THE MADRID ANTENNA COMPLEX .................................................................... 2 3. SIGNAL PROCESSING RESULTS .............................. 3 4. SUMMARY AND CONCLUSIONS .............................. 5 ACKNOWLEDGEMENTS .............................................. 6 Figure 1. Conceptual diagram of Swarm Array transmitter in orbit around Mars REFERENCES............................................................... 6 BIOGRAPHY ................................................................ 6 Our previous research [3] investigated the feasibility of a wideband Swarm Array in Martian orbit to address future communications requirements. Since 1. INTRODUCTION the uplink arraying technology from the Deep Space Two CubeSats, designated as Mars Cube One (MarCO) Network (DSN) to a target in space is well developed, A and B, were launched together with the InSight and has been operating reliably for many years, our spacecraft on May 5th, 2018, and accompanied the study considers the problem of the downlink from a InSight lander on its journey to Mars. As reported in a spaceborne swarm array as the dual of the DSN uplink previous paper [3], the combined performance of a arraying problem. We assumed the swarm array Swarm Array consisting of ~30 MarCO class CubeSats elements to be CubeSat-sized spacecraft, similar to configured as an orbiting phased-array can achieve MarCO. The main conclusion of the study was that a MRO (Mars Reconnaissance Orbiter) level high data rate downlink swarm array at Mars is feasible, performance, and with 95 MarCO CubeSats a Swarm and that approximately 30 MarCO CubeSat class Array could potentially achieve ten times MRO-level configured as a Swarm Array could achieve MRO-level performance, if phase-coherence can be maintained. performance, whereas 95 MarCO CubeSats could ideally achieve ten times MRO-level performance. The Swarm Array concept is illustrated in Figure 1, which shows a future Swarm Array in orbit around 978-1-5386-6854-2/19/$31.00 ©2019 IEEE 1 A conceptual model of the Swarm Array is shown in dynamics are typically known well enough to be Fig. 2. This model was used to generate the three- predicted a priori, leaving only small residual dimensional orbital dynamics of Fig. 3, both from the components that must be estimated in real-time. viewpoint of a “chief” spacecraft and the apparent view from Earth, over a timescale of days. distance to MarCO A: DSS-63 (blue); DSS-55 (red) 1.4 1.2 1 0.8 0.6 0.4 0.2 residual range to MarCO to A,meters residualrange b) 0 0 200 400 600 800 1000 1200 seconds Figure 4. a) Projected distance along the array beam line-of- sight; b) Madrid antenna range from MarCO A, with linear trend removed, showing approximately quadratic behavior on a short time-scale. Figure 2. Three-dimensional model of Swarm Array geometry. It can be seen that the residual range appears to be The simulated orbital trajectories of the Swarm array quadratic over the timescale of the data, similar to the are shown in Figures 3a, b and Figure 4a, where Figure maximum rate-of-change near the peaks of the 3a represents the view from the chief (or reference) projected distance from the Swarm Array along the spacecraft, Figure 3b is the earth-view and Figure 4a is line-of-sight, that must be removed to enable coherent the projected distance along the line-of-sight from the combining of the transmitted carriers at the target, as ground to the Swarm Array over two days, showing that described in [3]. inter-element spacing varies sinusoidally in a nominal Based on these observations, we postulate that earth- steady-state orbit. rotation causes qualitatively similar range variations of the residual antenna phase-centers as would be expected near the most challenging parts of the Swarm Array orbit, as shown in Figure 3c. The time-scale for the Swarm Array is periodic on a time-scale of about half a day, whereas the full-cycle variation of the Madrid antenna distances are periodic on a time-scale of one day, hence the results obtained here should be scaled to account for the different time-scales. Figure 3. Swarm Array orbital trajectories: a) viewed from the chief spaceraft; b) viewed from the Earth. 2. DATA ACQUISITION AT THE MADRID Analysis of the data in Figure 4a indicates that over ANTENNA COMPLEX short timescales of minutes, the relative delay between Three deep-space antennas of the Madrid Deep Space swarm elements remains nearly constant, with only a Network (DSN) complex recorded downlink data from small quadratic (and possibly higher order) component. MarCO A/B simultaneously on Day-of-Year 330 This observation will be used in the signal processing (DOY-330) of 2018, and this data was used for the analysis to validate the model for measuring and analysis reported in this paper. Six sets of complex predicting relative phase, in order to calibrate the baseband data were recorded on the wideband high- Swarm Array and keep it phased up even as the distance speed Open-Loop Recorder (OLR) of JPL’s Radio between array elements changes with time. Science group between 19:20 and 20:00 hours on DOY- 330. The recorded data was processed to determine the For comparison, the range between MarCO A and two feasibility of phasing up the array using real spacecraft of the Madrid antenna elements (DSS-63 and DSS-55) data obtained from deep space, having been generated has been plotted in Figure 4b over the time-interval of by MarCO A/B’s on-board transmitters, with the data. Linear trends have been subtracted out to characteristic phase-noise and trajectory dynamics reveal the higher order components, since orbital automatically embedded in the received signals. The 2 data consisted of an 18-minute segment of two-way expected due to the greater aperture of DSS-63. transmission from MarCO A recorded at 250 kHz sampling rate when the on-board oscillator was locked MarCO-A DSS-63/55/54 residual carriers MarCO-A DSS-63/55/54 derotated residual carriers 0 0 10 10 DSS-63 residual carrier DSS-63 derotated to an uplinked X-band reference signal, and a 24-minute residual carrier -1 DSS-55 -1 segment where both MarCO A and B oscillators were 10 DSS-54 10 DSS-55 DSS-54 free-running in one-way mode. However, after -2 10 10-2 examining the data it was decided to analyze only the -3 18 minute two-way data, because the one-way data was 10 10-3 of relatively poor quality and also contained numerous a) b) -4 10 10-4 -30 -25 -20 -15 -10 -5 0 5 10 -5 0 5 signal drop-outs. frequency, Hz frequency bins, Hz Figure 6. Expanded views of power spectra of MarCO data collected from three Madrid antennas: DSS-63/55/54. a) view of 3. SIGNAL PROCESSING RESULTS residual carrier near the nominal center frequency showing Two-way MarCO-A downlink data was recorded by the significant Doppler; b) counter-rotated and centered spectra. Radio Science Group’s OLR, at each of three DSN The counter-rotated residual carrier data were filtered to Madrid stations designated as DSS-63/55/54. Signal remove noise and interfering modulation components, processing algorithms were developed to process the and complex-downconverted to nominal baseband data, and it was shown that accurate phase-calibration resulting in relatively clean in-phase (blue) and can be obtained in a few seconds, then used to predict quadrature (red) signal components from all three future phase in order to maintain phase-coherence for antennas, as shown in Figure 7. Note that the amplitude the three antennas, which can also be viewed as proxies of the signal from the 70 meter antenna is roughly twice for a simulated Swarm Array due to reciprocity. In other as great (and less noisy) as the amplitudes of the 34 words, reciprocity implies that a single spacecraft meter antennas, hence the power is a factor of 4 greater, antenna in the vicinity of Mars (i.e. MarCO A) consistent with the previously observed power spectra. transmitting a signal to a cluster of three antennas on the MarCO-A DSS-63 IQ amplitudes MarCO-A DSS-55 IQ amplitudes MarCO-A DSS-54 IQ amplitudes ground rotating with the Earth’s surface, is qualitatively 10 10 10 8 real (I) Imaginary (Q) 8 8 similar to a single ground-based antenna transmitting a 6 6 6 s 4 s 4 real (I) imaginary (Q) 4 real (I) imaginary (Q) e e d 2 d 2 2 u u t t i i l signal to three Swarm Array element orbiting Mars.
Details
-
File Typepdf
-
Upload Time-
-
Content LanguagesEnglish
-
Upload UserAnonymous/Not logged-in
-
File Pages7 Page
-
File Size-