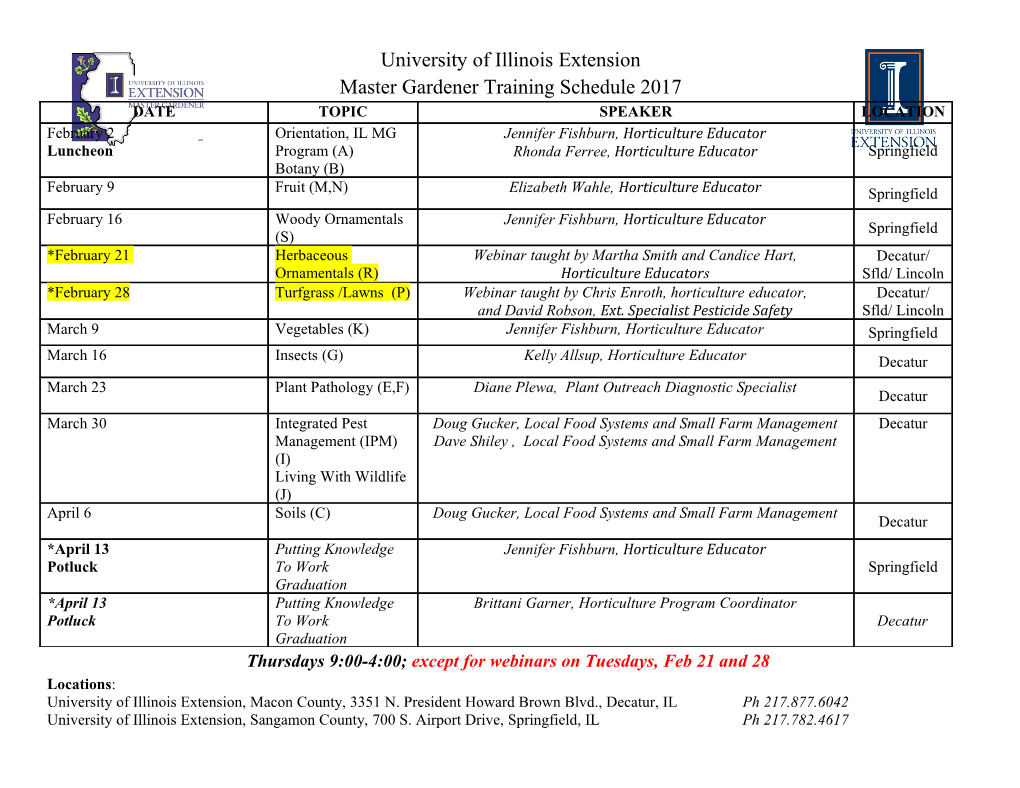
FACULTEIT WETENSCHAPPEN Opleiding Master of Science in de geologie Triggering mechanism and depositional processes of a late Holocene megaturbidite in Baker fjord, Chilean Patagonia Loïc Piret Academiejaar 2015–2016 Scriptie voorgelegd tot het behalen van de graad Van Master of Science in de geologie Promotor: Prof. Dr. S. Bertrand Begeleider: Prof. Dr. S. Bertrand Leescommissie: Dr. C. Kissel, Dr. M. Van Daele Cover image. Bathymetric map of the Baker/Martinez fjord complex. II ACKNOWLEDGEMENTS I was amazed by the amount of people that provided excellent help and guidance through the course of making this thesis and furthermore through my entire education. In this section I’d like to express my gratitude towards these people. First, I’d like to thank my promoter, Sébastien Bertrand (aka Seb). Your support and readiness to answer my questions are greatly appreciated. From the lab work in Paris, over figuring out how to exactly make a bathymetric map to teaching me how to properly write a scientific text, you gave me a great and educational experience. And most important, you guided me to become a young scientist. The sampling trip to Paris (Gif sur Yvette) was very pleasant and gave me some initial insight in the way of working and the larger context of the project. I would like to thank Catherine Kissel for her guidance and of course also for providing the magnetic data and radiocarbon dates. Also I would like to thank Camille Wandres for preparing the U-channels, making my lab work so much easier. All the people who provided essential data for this thesis are acknowledged: Ricardo de Pol Holz for the radiocarbon dates, Alvaro Tamayo for the foram data, Carlos Moffat & Lorena Rebolledo for the bathymetrical data, Xavier Morin of Ipev for the sub-bottom profiles. Greatly appreciated are the people of the RCMG for providing the educational software courses but also for the research seminars. These events fuelled my drive in research. There of course some RCMG scientists I’d like to thank in specific: Inka Meyer, you helped me a lot when there were problems in the lab and made those times very pleasant. Maarten Van Daele, thank you so much for helping me interpreting the seismics. Philipp Kempf, without you I wouldn’t have clue how old this turbidite was. Thanks for helping me with the age- depth modelling. A word of thanks goes out to Danielle Schram, thank you for your aid during my time in the lab. To my fellow students and friends, we shared a lot of great times in the classroom, on the field, after hours… And we also supported each other a lot. These past years were quite a fun and memorable experience thanks to all of you. I would further like to address two very important people to me: Mom and dad, thank you for providing me with the chance of education and for your endless support and love. Last but not least, Emma, thank you for motivating me and keeping me smile. Cheers, Loïc III TABLE OF CONTENTS 1. INTRODUCTION 1 1.1. STATE OF THE ART 1 1.2. OBJECTIVES & RESEARCH STRATEGY 2 2. REGIONAL SETTING 3 2.1. PATAGONIAN GEOLOGY 3 2.2. TECTONIC SETTING 3 2.3. CLIMATIC SETTING 5 2.4. THE BAKER /M ARTINEZ FJORD COMPLEX 6 3. MATERIALS & METHODS 8 3.1. CORE & SHIPBOARD DATA ACQUISITION 8 3.2. EARLIER AVAILABLE DATA 8 3.2.1. Shell Fragment Radiocarbon Dates 8 3.2.2. Foraminifera Abundance 8 3.2.3. Magnetic Data 9 3.3. CHRONOLOGY 9 3.3.1. Additional Radiocarbon Dating 9 3.3.2. Age-Depth Model 10 3.4. GRAIN -SIZE ANALYSIS 11 3.5. LOSS -ON-IGNITION (LOI) 11 3.6. BULK ORGANIC GEOCHEMISTRY 12 3.7. BATHYMETRIC MAPPING 13 4. RESULTS 14 4.1. BATHYMETRIC MAP 14 4.2. SUB -BOTTOM PROFILES 15 4.2.1. Profile A 15 4.2.2. Profile B 17 4.3. SEDIMENTOLOGICAL CHARACTERISATION 21 4.3.1. Core-to-Sub-Bottom Profile Correlation 23 4.4. BULK ORGANIC GEOCHEMISTRY 23 4.5. AGE – DEPTH MODEL 24 5. DISCUSSION 27 5.1. IDENTIFICATION OF THE BAKER FJORD MEGATURBIDITE & DEPOSITIONAL PROCESSES 27 5.1.1. Units A & B: Mass Transport Deposits (MTDs) 27 5.1.2. Units C & D: The Baker Fjord Megaturbidite 28 5.1.3. Unit E: Continuous Sedimentation 29 5.1.4. Upper limit of the Megaturbidite 29 5.1.5. Erosive features 30 5.1.6. Relation to the Baker Channel topography 31 5.2. AGE 31 5.3. TRIGGER OF THE BAKER FJORD MEGATURBIDITE 31 IV 5.3.1. Theoretical Aspects and Characteristic Features of different Turbidite Triggers 32 5.3.2. GLOF 33 5.3.3. Spontaneous Slope Failure 34 5.3.4. Seismic Trigger 34 5.4. IMPLICATIONS FOR PALEOCLIMATE RESEARCH IN BAKER FJORD 35 6. CONCLUSION 36 6.1. OUTLOOK 36 7. REFERENCES 38 8. DUTCH SUMMARY 48 9. APPENDIX 53 V VI 1. INTRODUCTION 1.1. State of the Art Megaturbidites worldwide have been the subject of many studies (Bourget et al., 2013; Fanetti et al., 2008; Haeussler et al., 2014; Polonia et al., 2013; Rothwell et al., 1998). These enormous subaqueous events can have catastrophic effects for fjord and lake ecology (e.g. water circulation & methane release) but also for the surrounding environment in the form of tsunami waves (Fanetti et al., 2008; Girardclos et al., 2006; Nisbet and Piper, 1998). Turbidite records are of key importance in studies of paleoseismic activity (Goldfinger et al., 2003; Moernaut et al., 2007; St-Onge et al., 2004; Strasser et al., 2006) and reconstructions of former (mega) flood events (Mulder et al., 2001; Russell and Knudsen, 1999; St-Onge and Lajeunesse, 2007; Zuffa et al., 2000). Climatic reconstructions and sea level changes can also be inferred from turbidite-bearing sedimentary archives (Blumberg et al., 2008; Henrich et al., 2010; Osleger et al., 2009; Pierau et al., 2010). Turbidites can be divided into two main classes, based upon their origin: Intrabasinal and extrabasinal (Zavala and Arcuri, 2016). Intrabasinal turbidites originate from and evolve completely within the aquatic basin whereas extrabasinal turbidites are generated by flooding events of nearby rivers and form hyperpycnal flows. Thus, three main potential triggers of turbidity flows can be defined: (1) Seismic trigger (intrabasinal); (2) Sediment overload, i.e., slope failure without seismic trigger (intrabasinal); (3) River flood (extrabasinal). Talling (2014) adds oceanographic processes (storm waves, tides and internal waves), volcanic eruptions and anthropogenic effects to this list and separates prevalent river floods from glacial outwash megafloods. To complete this list, sea level changes and gas hydrate destabilization have been recognised as turbidite triggers as well (Garcia-Castellanos et al., 2009; Puigdomenech et al., 2014; Rothwell et al., 1998). Turbidites that are an order of magnitude thicker are less frequent and generally called megaturbidites. Megaturbidites are not uncommon in Patagonia. Many of these large scale deposits occur in a deci- to metrical scale in both lake and fjord environments (Chapron et al., 2006; St-Onge et al., 2012; Van Daele et al., 2013). Since the term megaturbidite was introduced by Mutti et al. (1984), many disparate definitions have been proposed in the literature (Bouma, 1987; Lucchi and Valmori, 1980; Mutti et al., 1984; Reeder et al., 2000). However, all are in unison over the large quantity of sediment required for a turbidity flow deposit to be called megaturbidite. Also turbidites have been defined differently and definitions needed critical reviews and classifications (Mulder and Alexander, 2001; Shanmugam, 2000). The terms turbidity and density flows have been used confusingly as one and the same but a difference is made based upon sediment concentration (Mulder and 1 Alexander, 2001). Subaqueous sediment flows where fluid turbulence forms the dominant particle-support mechanism are called turbidity flows whereas density flows constitute the ones with grain to grain support. For the purpose of this thesis, turbidity flows will be used henceforth. The Patagonian fjords form an area where the three potential triggers mentioned above (earthquake, slope failure, flood) are all equally possible, as the area is particularly sensitive to both climate changes and seismic activity. A likely trigger in the region is a glacial lake outburst flood (GLOF, or jökulhlaups). A GLOF is defined as a sudden release of lake water that was previously dammed by a glacier. They are extreme water discharge events that carry enormous amounts of sediment (reaching the Baker fjord) which eventually could lead to (flood-induced) event deposits (Quiroga et al., 2012; Worni et al., 2014). Their frequency and magnitude have been linked to (current) climate change (Kargel et al., 2012) and these events frequently occur in Patagonia (Dussaillant et al., 2010). Due to the proximity of the study area close to an active fault system (see specified section 2.2), seismic triggering could likewise have caused mass transport deposits. Examples of these have been found in other Patagonian fjords (e.g. St-Onge et al., 2012; Van Daele et al., 2013). Finally, high sedimentation rates (especially in ice proximal environments) and the relatively steep slopes that generally characterize fjords (Howe et al., 2010) facilitate turbidite triggering by sediment overload, i.e. the “spontaneous” trigger. This study fits in the growing number of research projects focusing on the area’s geology, oceanography, climate etc. (Pantoja et al., 2011). It makes use of fjords sediments, which are increasingly recognized in a variety of studies as excellent recorders of climate and environmental change (see review of Howe et al., 2010). 1.2. Objectives & Research Strategy The study object of this master dissertation is a megaturbidite found in core MD07-3121 (Baker fjord, Chile, 48° S) during the PACHIDERME research cruise onboard R/V Marion Dufresne (Kissel, 2007a).
Details
-
File Typepdf
-
Upload Time-
-
Content LanguagesEnglish
-
Upload UserAnonymous/Not logged-in
-
File Pages65 Page
-
File Size-