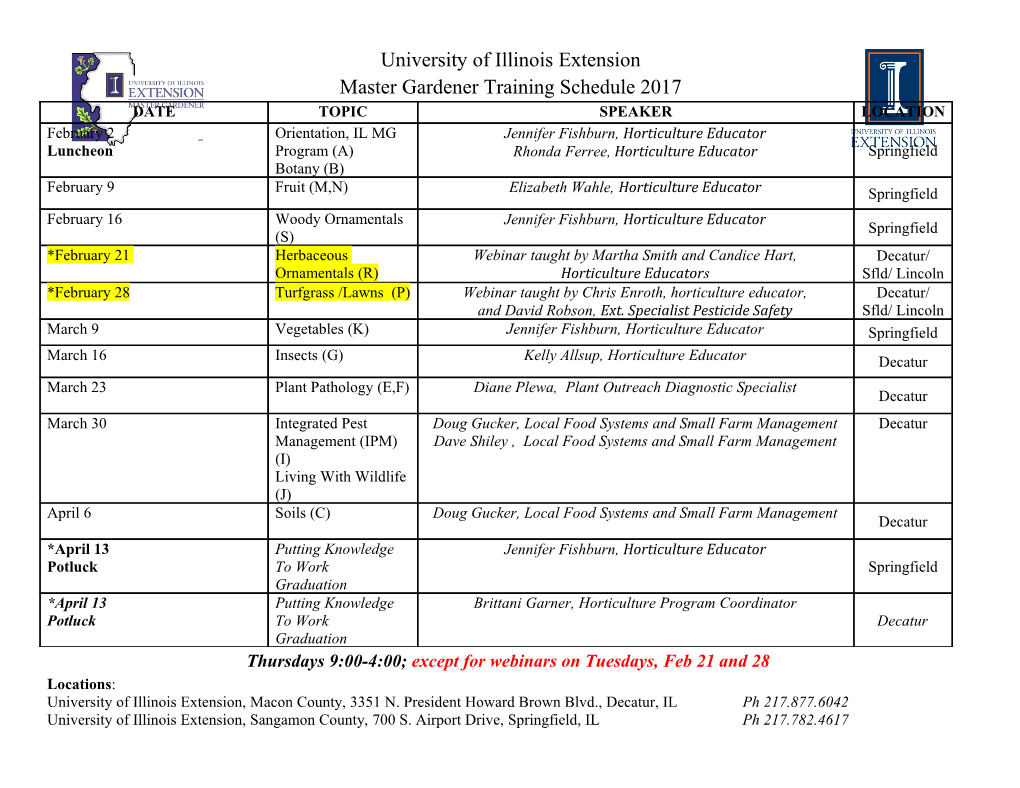
Hindawi Mathematical Problems in Engineering Volume 2020, Article ID 8614841, 9 pages https://doi.org/10.1155/2020/8614841 Research Article Degenerate Analogues of Euler Zeta, Digamma, and Polygamma Functions Fuli He ,1 Ahmed Bakhet ,1,2 Mohamed Akel,3 and Mohamed Abdalla 3,4 1School of Mathematics and Statistics, Central South University, Changsha 410083, China 2Department of Mathematics, Faculty of Science, Al-Azhar University, Assiut 71524, Egypt 3Department of Mathematics, Faculty of Science, South Valley University, Qena 83523, Egypt 4Department of Mathematics, College of Science, King Khalid University, Abha 61471, Saudi Arabia Correspondence should be addressed to Mohamed Abdalla; [email protected] Received 23 February 2020; Accepted 7 April 2020; Published 6 May 2020 Guest Editor: Praveen Agarwal Copyright © 2020 Fuli He et al. (is is an open access article distributed under the Creative Commons Attribution License, which permits unrestricted use, distribution, and reproduction in any medium, provided the original work is properly cited. In recent years, much attention has been paid to the role of degenerate versions of special functions and polynomials in mathematical physics and engineering. In the present paper, we introduce a degenerate Euler zeta function, a degenerate digamma function, and a degenerate polygamma function. We present several properties, recurrence relations, infinite series, and integral representations for these functions. Furthermore, we establish identities involving hypergeometric functions in terms of de- generate digamma function. 1. Introduction framework have been widely considered in the literature, for instance, [24–27]. (e gamma, digamma, and polygamma functions have an In this section, we present some basic properties and increasing and recognized role in fractional differential well-known results on a degenerate gamma function which equations, mathematical physics, the theory of special we need in this work. In Section 2, we introduce a degenerate functions, statistics, probability theory, and the theory of Euler zeta function and discuss its region of convergence, infinite series. (e reader may refer, for example, to [1–9]. integral representation, and infinite series representation. In (ese functions are directly connected with a variety of Section 3, we define a degenerate digamma function along special functions such as zeta function, Clausen’s function, with its region of convergence and integral representation. and hypergeometric functions. (e evaluations of series We also give certain recurrence relations and formulae involving Riemann zeta function ζ(s) and related functions satisfied by the degenerate digamma function. In Section 4, have a long history that can be traced back to Christian we define a degenerate polygamma function and describe its Goldbach (1690–1764) and Leonhard Euler (1707–1783) convergence conditions. Some recurrence relations satisfied (see, for details, [10]). (e Euler zeta function and its by the degenerate polygamma function are also given here. generalizations and extensions have been widely studied Finally, in Section 5, the hypergeometric functions are [11–15]. expressed in terms of the degenerate digamma function. ∗ Later on, these functions arise in the study of matrix- In [26], a degenerate gamma function, denoted Γλ , has valued special functions and in the theory of matrix-valued been defined by orthogonal polynomials, see e.g., [16–23] and the references ∞ therein. ∗ − t/λ z− 1 Γλ (z) � Z (1 + λ) t dt; λ ∈ (0; 1); Re(z) > 0: Motivated by this great importance of these functions, 0 their investigations and generalizations to the degenerate (1) 2 Mathematical Problems in Engineering (e basic results of this function, given in [26], can be 2. Degenerate Euler Zeta Function summarized in the following lemma. (e Euler zeta function in two complex variables s; z such that Re(s) > 0 and Re(z) > 0 is defined by (see [12, 24]) ( ; ) z C Lemma 1. Let λ ∈ 0 1 . 1en, for ∈ with ∞ n (z) ; ∗(z) (− 1) Re > 0 Γλ satisfies ζ (s; z) � 2 X : (7) E (n + z)s λz n�0 Γ∗(z + 1) � Γ∗(z); λ log(1 + λ) λ An integral representation of ζE(s; z) is given as ∞ λ − 1 s− 1 Γ∗(1) � ; ζE(s; z) � Γ (s) Z F(− t; z)t dt; (8) λ log(1 + λ) 0 (2) where 2ezt ∞ tn k+1z(z − )···(z − k) F(t; z) � � X E (z) ; (9) ∗ λ 1 ∗ + et n n! Γ (z + 1) � Γ (z − k); k ≥ 0; 1 n�0 λ (log(1 + λ))k+1 λ (3) where En(z) is the Euler polynomial of degree n. When z � 0, En � En(0) are Euler numbers (see, [12, 14]). Kim in k+1 [14] obtained that ζn(− n; z) � En(z); n ≥ 0. ∗ λ k! Γ (k + 1) � ; k ∈ N: (4) In this section, we consider a degenerate analogue of the λ ( ( + ))k+1 log 1 λ Euler zeta function which is given as ∞ Also, we can easily show that − 1 s− 1 ζE (s; z) � Γ (s) Z Fλ(− t; z)t dt; (10) λ 0 ∗ Corollary 1. Let λ ∈ (0; 1). 1en, Γλ (z) satisfies where λ ∈ (0; 1); s; z ∈ C with Re(s) > 0; Re(z) > 0, and z ∞ n ∗ λ 2 zt t Γ (z) �"# Γ(z); z ∈ C; Re(z) > 0; (5) F (t; z) � (1 + λ) /λ � X Eλ(z) : (11) λ log(1 + λ) λ t/λ n n! 1 +(1 + λ) n�0 where Γ(z) is the gamma function. Moreover, for m; n ∈ N, By (9) and (11), it follows that we have n ∗ ∗ ∗ λ λ (m) (n) � B(m; n) (m + n); ( ) E (z) � ! En(z); (12) Γλ Γλ Γλ 6 n ln(1 + λ) B(:; :) where is the beta function. which is the degenerate Euler polynomial of degree n. From (10) and (11), we obtain that ∞ ∞ ∞ − 1 s− 1 − 1 m − (m+z)t/λ s− 1 Γ (s) Z Fλ(− t; z)t dt � Γ (s) Z 2 X (− 1) (1 + λ) t dt 0 0 m�0 ∞ ∞ s− 1 − m − τ � 2Γ 1(s) X (− 1) Z (1 + λ) τ/λ dτ (13) (m + z)s m�0 0 Γ∗(s) ∞ 1 � 2 λ X (− 1)m : (s) (m + z)s Γ m�0 s (us, using (10) and (13), we conclude the following (s; z) � (s; z) λ ; ( ) ζE ζE �ln(1+λ) � 15 result. λ where ζE(s; z) is the Euler zeta function defined by (7). Theorem 1. For s; z ∈ C with Re(s) > 0; Re(z) > 0, and Furthermore, from (11), it follows λ ∈ (0; 1), the degenerate Euler zeta function ζ (s; z) defined Eλ ∞ ∞ (− 1)m ∞ in (10) has the following infinite series representation: Γ− 1(s) Z F (− t; z)ts− 1dt � Γ− 1(s) X Eλ(z) Z ts+m− 1dt: λ n m! ∗ ∞ 0 m�0 0 Γλ (s) m 1 ζE (s; z) � 2 X (− 1) s: (14) λ (s) (m + z) (16) Γ m�0 Moreover, in view of (5), we have Hence, we obtain the following results. Mathematical Problems in Engineering 3 Theorem 2. For s; z ∈ C with Re(s) > 0; Re(z) > 0, and ∞ z − 1 (z) � − c + ; λ ∈ (0; 1), the degenerate Euler zeta function ζ (s; z), de- ψ P (25) Eλ n�0 (n + 1)(n + z) fined in (10), satisfies ∞ m ∞ where − 1 λ (− 1) s+m− 1 n ζE (s; z) � Γ (s) X En(z) Z t dt: (17) 1 λ m! 0 c � lim 0@X − log n1A � − 0:577215 (26) m�0 n⟶∞ k k�1 And for n ∈ N ∪ f0 g, n is the Euler–Mascheroni constant. Hence, substituting (25) 2πi(− 1) λ λ ζE (− n; z) � E � E (z): (18) into (24), one gets the following. λ n!Γ(− n) n n Theorem 4. For z ∈ C; Re(z) > 0, and λ ∈ (0; 1), Remark 1. Note that ζE (s; z) is an entire function in the λ n 1 λ ∗(z) � − 24 n − P 35; complex s− plane. ψλ log ! limn⟶∞ log log(1 + λ) j�0 z + j (27) Remark 2. lim ζE (− n; z) � En(z) � ζE(− n; z): ( ) ∞ λ⟶0 λ 19 ∗ λ 1 ψλ (z) � log ! − c +(z − 1) P ; log(1 + λ) n�0 (n + 1)(n + z) (28) 3. Degenerate Digamma Function ∞ (e digamma function, denoted by ψ(z), is the logarithmic ∗ λ 1 ψλ (z + 1) � log ! − c + z P : (29) derivative of the gamma function given by [6, 16, 28]: log(1 + λ) n�1 n(n + z) d Γ′(z) ∗(z) ψ(z) � log Γ(z) � : (20) Next, the degenerate digamma function ψλ defined dz Γ(z) by (21) can be expressed as a series expression in terms of Riemann’s zeta function. Using In this section, we define a degenerate digamma function ∞ m − − − z as follows: (n + z) 1 � n 1 X � � ; (30) n m�0 d Γ∗′ (z) ψ∗(z) � log Γ∗(z) � λ ; (21) λ λ ∗ equation (29) can be rewritten as dz Γλ (z) λ ∞ ∞ where Γ∗(z) is the degenerate gamma function defined by ψ∗(z + 1) � log ! − c − X X n− (m+1)(− z)m: λ λ ( + ) (1). Now, we are going to obtain certain functional equations log 1 λ n�1 m�1 ∗ involving the degenerate digamma function ψλ (z). Using (31) (2) and (21), it follows that (us, one gets the following. Γ∗′(z + 1) zΓ∗(z) �′ ∗(z + ) � λ � λ ψλ 1 ∗ ∗ Theorem 5. For z ∈ C; Re(z) > 0, and λ ∈ (0; 1), Γλ (z + 1) zΓλ (z) ∞ ∗ λ m ′ ∗ (22) ψλ (z + 1) � log ! − c − X ζ(m + 1)(− z) : Γλ (z) 1 ∗ 1 ( + ) � + � ψ (z) + ; log 1 λ m�1 Γ∗(z) z λ z λ (32) Re(z) > 0: Note that these series converge absolutely for jzj < 1.
Details
-
File Typepdf
-
Upload Time-
-
Content LanguagesEnglish
-
Upload UserAnonymous/Not logged-in
-
File Pages9 Page
-
File Size-