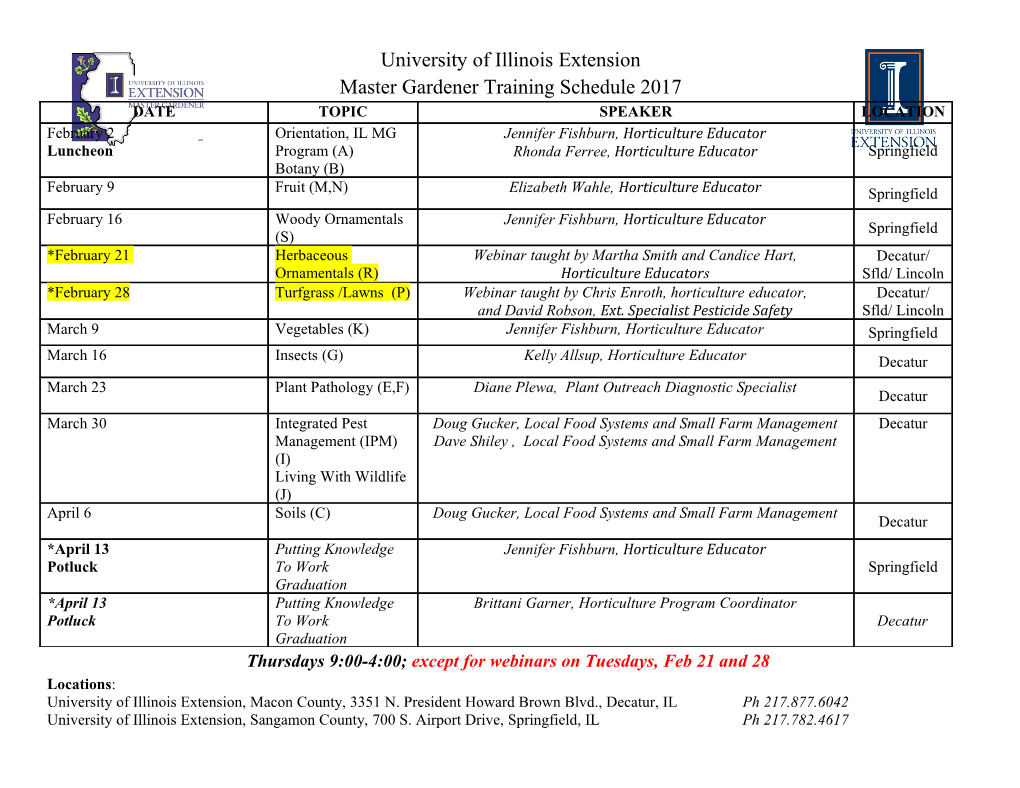
Florida State University Libraries Electronic Theses, Treatises and Dissertations The Graduate School 2011 New Materials Grown from Ca/Li Flux David A. Lang Follow this and additional works at the FSU Digital Library. For more information, please contact [email protected] THE FLORIDA STATE UNIVERSITY COLLEGE OF ARTS AND SCIENCES NEW MATERIALS GROWN FROM CA/LI FLUX By DAVID A. LANG A Thesis submitted to the Department of Chemistry and Biochemistry in partial fulfillment of the requirement for the degree of Master of Science Degree Awarded: Summer 2011 The members of the committee approve the thesis of David Lang defended on June 22, 2011. _______________________________________ Susan Latturner Professor Directing Thesis _______________________________________ Albert E. Stiegman Committee Member _______________________________________ Oliver Steinbock Committee Member Approved: _____________________________________ William Cooper, Associate Chair, Department of Chemistry and Biochemistry The Graduate School has verified and approved the above-named committee members. ii TABLE OF CONTENTS List of Tables iv List of Figures v Abstract vi Chapter 1: Introduction to flux synthesis 1 1.1 Metal flux 1 1.2 Alkaline earth/alkali metal fluxes 2 1.3 Expected products of synthesis in Ca/Li flux 5 1.3.1 Zintl phases 5 1.3.2 Intermetallics 6 1.3.3 Hydrogen storage materials 7 1.4 Characterization of products 7 1.4.1 Elemental analysis 8 1.4.2 X-ray diffraction 8 1.4.3 NMR 9 1.4.4 Protolysis, hydriding and dehydriding 10 1.4.5 Band structure calculations 10 Chapter 2: LiCa2C3H 11 2.1 Introduction 11 2.2 Experimental procedure 11 2.3 Results and discussion 15 Chapter 3: LiCa7Si3H3 23 3.1 Introduction 23 3.2 Experimental procedure 23 3.3 Results and discussion 25 3.4 Thermo-volumetric measurements 31 Chapter 4: LiCa7Ge3H3 and LiCa11Ge3OH4 32 4.1 Introduction 32 4.2 Experimental procedure 32 4.3 Results and discussion 35 4.4 Future work 40 Chapter 5: Conclusions and future work 41 5.1 Ca/S/C 41 5.2 Ca/B/C 43 References 45 Biographical sketch 48 iii LIST OF TABLES 2.1. Data collection and structure refinement parameters for LiCa2C3H 13 2.2. Atomic coordinates, site occupancy factors (s.o.f.), and thermal displacement parameters for LiCa2C3H 13 2.3. Selected interatomic distances (Å) in the LiCa2C3H structure 14 3.1. Crystallographic data collection parameters for LiCa7Si3H3 24 3.2. Atomic position and thermal displacement parameters for LiCa7Si3H3 24 3.3. Bond lengths observed for LiCa7Si3H3 25 4.1. Selected crystal data and structural refinement parameters for LiCa7Ge3H3 and LiCa11Ge3OH4 34 4.2. Atomic coordinates and thermal displacement parameters for LiCa7Ge3H3 34 4.3. Atomic coordinates and thermal displacement parameters for LiCa11Ge3OH4 35 4.4. Comparison of selected interatomic distances in LiCa7Ge3H3 and LiCa11Ge3OH4 37 iv LIST OF FIGURES 1.1. A representative depiction of metal crucibles and their contents after centrifugation 2 1.2. The Ca/Li phase diagram. The circle highlights the 50:50 Ca/Li mixture and its melting point of 300°C 3 1.3. Heating profile commonly used for Ca/Li flux reactions 4 1.4. Calcium distillation apparatus 5 1.5. Vial on the right contains calcium metal before distillation; vial on the left is filled with Ca after distillation 5 2.1. A crystal of LiCa2C3H under paratone oil 16 2.2. Powder X-ray diffraction patterns for products of Ca/Li/C/CaH2 reactions with reactant mmol ratios of 10:10:6:x 16 2.3. Structure of LiCa2C3H 17 7 2.4. Li MAS NMR spectra of LiCa2C3H samples synthesized using Ca/Li/C/CaH2 mmol ratios of 10:10:6:x 20 2.5. 13C MAS-NMR spectrum 20 1 2.6. H MAS-NMR spectrum of LiCa2C3H 21 2.7. Total and partial density of states for LiCa2C3H 22 3.1. SEM micrograph of a crystal of LiCa7Si3H3 26 3.2. Structural variants of Ca3Ni3Si2 26 3.3. Structure of LiCa7Si3H3 27 3.4. View of hydride layer in LiCa7Si3H3 (viewed down the b-axis), and coordination environment of the three hydride sites 28 3.5. MAS NMR spectra of LiCa7Si3H3 30 3.6. Hydride decompositions of LiAlH4 standard material are shown by the 2 spikes in pressure that are marked 31 4.1. Powder X-ray diffraction of Ca/Li/Ge/CaH2/CaO reactions. 36 4.2. The LiCa11Ge3OH4 structure, viewed down the c-axis 38 4.3 The LiCa11Ge3OH4 structure, highlighting the locations and connectivity of the hydride-centered octahedra (yellow) 39 4.4. Density of states diagram for LiCa7Ge3H3 40 5.1. Structure of Ca12S(C3)2(CxH1-x)4 42 5.2. Structure of (Ca/Li)15(CBC)6(C2)2(C)x 44 v ABSTRACT Molten metal fluxes are useful in materials synthesis. Dissolution in molten metal activates reactants at temperatures well below their melting point. Lower temperatures and the modified energetics in a flux increase the possibility of isolation of complex metastable or kinetically stabilized phases. In contrast to the powders often obtained by conventional solid state synthesis methods, the solution phase character of flux reactions promotes the growth of crystals, which are required for accurate structural and electronic characterization. Ca/Li flux solvates lightweight refractory elements such as carbon and boron. It also dissolves many ionic compounds such as CaH2, Ca3N2, and LiF. This allows for synthesis of phases ranging from strongly delocalized intermetallics to complex salts. In particular, dissolution of CaH2 makes Ca/Li flux a very promising medium for growth of new metal hydride phases. These hydride compounds may have great potential as hydrogen storage materials. The ionic products such as Zintl and complex salt phases have interesting electronic properties because these classes of compounds are usually semiconductors. Reactions of light main group elements (B, C, Si) and ionic compounds (CaH2, CaO, Li3N) have been carried out in Ca/Li flux. Several Zintl phase hydrides including LiCa2C3H and LiCa7Si3H3 have been produced. These compounds are of interest because of their new crystal structures and their release of H2 gas in decomposition reactions. Structures are discussed in detail to explain the different bonding environments in these compounds. Efforts to explore and eliminate the oxide and hydride contaminants in the reactive flux have also netted interesting results. A new boride carbide was found using distilled flux. vi CHAPTER 1 FLUX SYNTHESIS 1.1 Metal flux synthesis A major contribution to advances in the study of intermetallic and Zintl phases is the development of new synthetic methods. Traditionally, reactants are combined in specific ratios to target specific phases. The reactants are ground and pressed into a pellet, which is heated at very high temperatures. The product of such reaction is generally isolated in powder form and rarely pure after one cycle; several cycles of grinding and heating are often required. The main disadvantage of this synthetic method is that it favors thermodynamically driven products, which are often binary phases or simple ternary phases. As an alternative to traditional methods, an appropriate flux can be carefully chosen to dissolve reactants at much lower temperatures than typically used for solid-state synthesis.1,2,3 This will enhance diffusion and favor kinetically stable products because it allows reactants to find a low temperature product with local energy minimum structure. This will eliminate unwanted thermodynamically driven products like binary phases and simple ternary phases. Additionally, the liquid state of reaction will promote the growth of crystals making the products easier to analyze and molten flux is in many cases easily removed leaving the pure product. For a metal to be a good choice as a flux for reaction chemistry it should melt and dissolve reactants at reasonably low temperatures so that normal heating equipment and containers can be used, and it must allow separation of the excess flux from the products. Additionally, it is important for the reactants to have reasonable solubilities in the flux to avoid exceedingly long growth times.4 While many metals have very high melting points, eutectic combinations of different metals often have melting points well below the temperatures of classical solid-state synthesis. In a reactive flux components act not only as solvents, but also as reactants, providing species that can be incorporated into the final product.5 Flux and reactants are combined in a crucible, which is placed into a fused silica tube and sealed under vacuum. The reaction tubes are placed in a furnace and the heating profile is chosen based on the melting point of the flux and the solubility of the reactants in it. The temperature needs to be somewhat elevated to dissolve the refractory elements, such as carbon. When the reactions are done excess flux is removed, while it is still liquid, by centrifuging and the product is isolated (see figure 1.1). 1 Figure 1.1. A representative depiction of metal crucibles and their contents after centrifugation. Crystalline products adhere to the reaction end of crucible (left), and flux melt is decanted to the other end (right). The crucible material is determined by the flux that is being used. A crucible should be resistant to corrosion by the solvent. As a general rule suitable crucible materials have different bonding from that of the flux solvent and the other reactants that are used in the synthesis. A large difference in the chemical bond type leads to sufficiently small solubility of the crucible material in the flux solvent, especially when the material has a high melting point. For instance, platinum crucibles are often recommended for oxide melts, since the ionic reactants will not attack the Pt vessel. Additionally, strength of the crucible needs to be high enough to contain pressures from the solution and any gas present or generated at the high temperatures at which the synthesis takes place.
Details
-
File Typepdf
-
Upload Time-
-
Content LanguagesEnglish
-
Upload UserAnonymous/Not logged-in
-
File Pages55 Page
-
File Size-