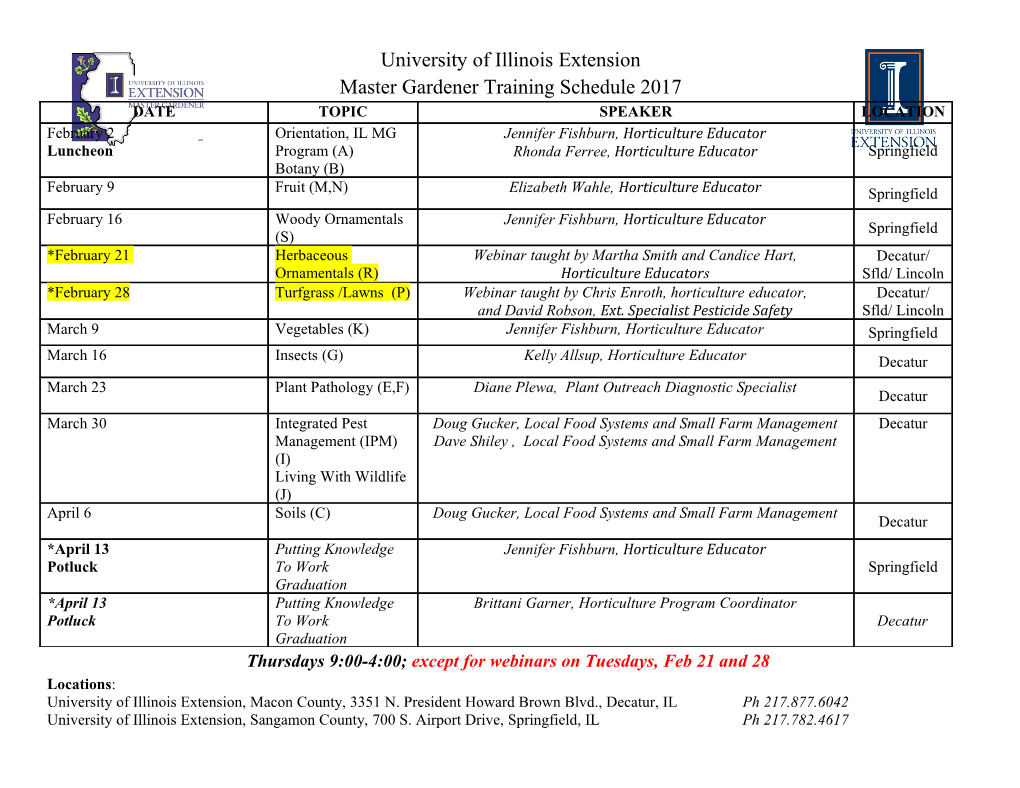
Limnol. Oceanogr., 32(4), 1987, 8 15-824 0 1987, by the American Society of Limnology and Oceanography, Inc. Big Soda Lake (Nevada). 4. Vertical fluxes of particulate matter: Seasonality and variations across the chemocline James E. Cloern, Brian E. Cole, and Sally M. Wienke U.S. Geological Survey, 345 Middlefield Road, Menlo Park, California 94025 Abstract Vertical fluxes of particulate organic matter were measured with sediment traps above and below the chcmocline of Big Soda Lake to dcfinc the seasonality of sinking losses from the mixolimnion and dctcrmine the effectiveness of the chcmocline (pycnocline) as a barrier to the sinking of biogenic particles. Seasonality of sedimentation rates reflected seasonal changes in the community of au- totrophs. During summer-autumn, when production is dominated by autotrophic bacteria, vertical fluxes were small: z 100 mg C m-* d-l and ~0.5 mg Chl a m-* d-r. Following the winter diatom bloom, vertical fluxes increased markedly: ~570 mg C m-* d-l and 23 mg Chl a m-* d-r. The bulk of the seston (> 80%) and particulate carbon (= 65%) sinking to the chemocline passed through it, showing that this very sharp density discontinuity dots not effectively retard the sinking of particulate matter. However sinking losses of particulate carbon were generally small (= 10%) relative to previous measures of primary productivity, indicating that the mixolimnion is a zone of efficient carbon cycling. Exceptions occurred following the winter bloom when sinking losses were a larger fraction (= 40%) of productivity. Because density discontinuities inhibit lakes. If the chcmocline is an inefficient fil- vertical mixing, a potential function of the ter, then the monimolimnion can be a sink chemocline (pycnocline) in meromictic lakes for nutrients and organic matter; if the che- is to cause the vertical flux of particulate mocline is an efficient filter, then nutrient organic matter (POM) to be unidirectional cycling in the mixolimnion is similar to that and downward. Particles sinking to the low- in holomictic lakes. er layer (monimolimnion) will be trapped The first objective of this study was to and either buried in the sediments or de- estimate the filtering efficiency of the che- composed. The only mechanisms for rc-en- mocline in Big Soda Lake. It was done by try into the upper layer (mixolimnion) are measuring sinking fluxes of POM just above through bubble ebullition of gaseous de- and below the chemocline, which is an cx- composition products (e.g. methane) or very treme density discontinuity (42 kg m-3: slow diffusion across the chemocline. Under Kimmel et al. 1978) that allows a robust this circumstance, a significant fraction of test of the hypothesis that density differ- primary production in the trophogenic mix- ences retard the sinking of biogenic parti- olimnion may be lost to the monimolim- cles. A second objective was to determine nion, and an important mechanism of nu- the seasonality of vertical fluxes to the che- trient regeneration is absent from the surface mocline and relate them to seasonal changes layer. in the pelagic autotrophs. During summer- The chemocline may also be the site of autumn when the mixolimnion is thermally localized accumulations of seston (Culver stratified, phytoplankton biomass is low and and Brunskill 1969; Hamner et al. 1982; autotrophic production is dominated by Cloern et al. 1983a), suggesting that it re- photosynthetic and chemosynthetic bacte- tards the sinking of biogenic particles. If the ria localized in a layer near the oxycline (see chemocline is a perfectly efficient “filter” for figure 2: Zehr et al. 1987). During winter sinking POM, then it may be an active site mixing the bacterial layer is disrupted but of nutrient regeneration and decomposition ammonia is brought to the surface and stim- for POM produced in the trophogcnic zone ulates a phytoplankton bloom dominated (Culver and Brunskill 1969). Hence, the ef- by the pennate diatom Nitzschia palea. Our ficiency of the chemocline as a filter for sink- study was designed around this annual cycle ing seston influences the fate of organic mat- and included measurements during the an- ter produced autotrophically in meromictic ticipated period of the winter diatom bloom, 815 816 Cloern et al. the spring transition period, and the sum- unscreened sample and aliquots after mer-autumn period of low phytoplankton screening with a 60-pm Nitex mesh. biomass. The contents of the BOD bottles, water We thank A. Alpine and C. Culbertson samples, and material from sediment traps for their assistance, and J. Zehr and R. Smith were also analyzed for photosynthetic pig- for manuscript reviews. ments (Chl a and Bchl a). Replicate aliquots were collected on 47-mm GF/AE filters and Methods frozen. In the laboratory they were ground Sampling was done over the deepest part with 90% acetone and extracted overnight of the lake in July and October 1984 and in in a refrigerator. Concentrations were dc- February and May 1985. Vertical profiles termined spcctrophotometrically with the (to 30 m) of temperature and dissolved oxy- pheopigment correction of Lorenzen (1967) gen (DO) were obtained with an Orbisphere for Chl a and the equation of Takahashi and Laboratories thermistor and DO sensor. Ichimura (1970) for Bchl a. Figure 1 com- Water samples were collected at 1O-l 5 pares absorption spectra of pigment extracts depths with a 2.2-liter PVC Kemmerer bot- taken in the aerobic epilimnion (phyto- tle; sample depths were chosen on the basis plankton) and in the photosynthetic bacte- of temperature and DO profiles to ensure rial (PSB) layer. Since Bchl a is calculated sampling around the thermocline and oxy- only from the absorbance peak at 772 nm Cline. Samples were always taken at the and algal pigments do not absorb at this depths of sediment trap deployment (30 m, wavelength (see Fig. In), calculation of Bchl 40 m) and at the chemocline (34.5 m). Water a was straightforward even for water sam- samples were placed in opaque containers, ples containing both phytoplankton and chilled on ice, and processed within 12 h of thcsc photosynthetic bacteria. collection. However, Bchl a interferes with the de- After sampling, replicate sediment traps termination of algal Chl a. Calculation of were placed above (30 m) and below (40 m) Chl a includes subtraction of a turbidity the chemocline. The traps were PVC tubes blank at 750 nm, typically with very small (65 cm long, 7.5-cm diam) suspended from values (Strickland and Parsons 1972). Ace- a buoyed and anchored line. Rates of POM tone extracts of PSB from Big Soda Lake decomposition were measured with repli- absorb strongly at 750 nm (Fig. IB), and the cate 150-ml BOD bottles that were filled Lorenzen (1967) equation could not be ap- with 30- or 40-m water and attached to the plied directly to samples containing PSB. line at the depth of collection. After 6-10 To calculate Chl a in anoxic waters, we sub- d, sediment traps and BOD bottles were re- tracted the value 0.69 X A772 from the mea- covered, and their contents were drained sured absorbance at 750 nm, where A,,, is into containers and processed immediately. the absorbance at 772 nm. The constant Concentrations of particulate carbon (PC) 0.69 defines the PSB contribution to absor- and particulate nitrogen (PN) were mea- bance at 750 nm and was determined from sured in water samples collected with the the mean value of the ratio A750 : A772 for Kemmerer bottle as well as those from the all samples collected in anoxic waters. sediment traps and BOD incubations. Ali- Aliquots of each sediment trap were pre- quots were filtered on precombusted 13-mm served in Lugol’s solution for microscopic GF/AE filters that were loaded into nickel examination. The remaining contents of capsules and dried in a desiccator. They each trap wcrc used to measure the total were later analyzed for PC and PN with an mass of seston collected during the deploy- elemental analyzer (Perkin-Elmer model ment period. We did this gravimetrically by 240B) with acetanilide as a standard. Rep- collecting sediment on Nuclepore filters (0.2 licate aliquots were analyzed from the scd- pm) and then reweighing them after air- iment traps and BOD bottles. Particulate drying. carbon collected in the sediment traps was PC, PN, and pigment profiles of the water size-fractionated by analyzing aliquots of column were used to calculate total standing Big Soda Lake: Sedimentation 817 stocks by depth integration (O-30 m) with trapezoidal quadrature. Vertical fluxes of PC, seston, and pigments were calculated from measured concentration changes in the sediment traps and BOD bottles. We as- sumed that two processes determine the ac- cumulation rate of biogenic seston in sedi- ment traps: decomposition (or production) of POM during the deployment period, and sedimentation. These processes are defined bY dC/dt = rC + G, (1) where C is the concentration of a seston constituent (PC, Bchl a, Chl a), t is time, r is the specific rate constant of decomposi- Photosynthetic Bacteria tion for constituent C, and G is the accu- mulation rate from sinking of constituent C. The solution to Eq. 1 yields a measure of the sedimentation rate (mg m-3 d--l) cor- 0’ L--JI I I I 1 I -II rected for decomposition (see Ducklow et 650 700 750 800 al. 1982): Wavelength (nm) Fig. 1. Typical absorption spectra of acetone ex- G = r[C,. - C,exp(rT)]l[exp(rT)-- 11, (2) tracts taken from samples collected (A) in the cpilim- where r is the specific rate of decomposition nion of Big Soda Lake (showing the algal Chl a peak at 665 nm), and (B) in the PSB layer (showing the Bchl (d-l), C,.
Details
-
File Typepdf
-
Upload Time-
-
Content LanguagesEnglish
-
Upload UserAnonymous/Not logged-in
-
File Pages10 Page
-
File Size-