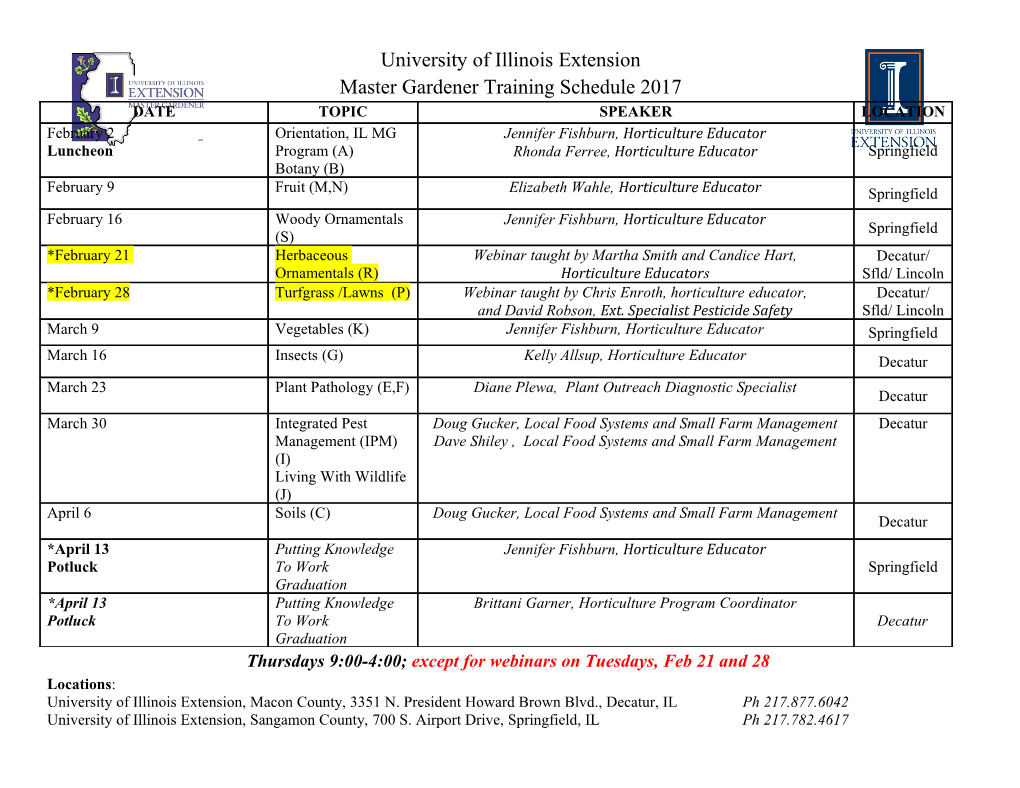
International Journal of Molecular Sciences Review CRISPR/Cas Derivatives as Novel Gene Modulating Tools: Possibilities and In Vivo Applications 1,2, 1,2,3, 2,4 2,4 Xingbo Xu y, Melanie S. Hulshoff y, Xiaoying Tan , Michael Zeisberg and Elisabeth M. Zeisberg 1,2,* 1 Department of Cardiology and Pneumology, University Medical Center Göttingen, Robert-Koch-Str. 40, 37075 Göttingen, Germany; [email protected] (X.X.); melanie.hulshoff@med.uni-goettingen.de (M.S.H.) 2 German Centre for Cardiovascular Research (DZHK), 37075 Göttingen, Germany; [email protected] (X.T.); [email protected] (M.Z.) 3 Department of Pathology and Medical Biology, University Medical Center Groningen, Hanzeplein 1, 9713 GZ Groningen, The Netherlands 4 Department of Nephrology and Rheumatology, University Medical Center of Göttingen, Robert-Koch-Str. 40, 37075 Göttingen, Germany * Correspondence: [email protected] These authors contributed equally to this work. y Received: 2 April 2020; Accepted: 23 April 2020; Published: 25 April 2020 Abstract: The field of genome editing started with the discovery of meganucleases (e.g., the LAGLIDADG family of homing endonucleases) in yeast. After the discovery of transcription activator-like effector nucleases and zinc finger nucleases, the recently discovered clustered regularly interspaced short palindromic repeats (CRISPR)/CRISPR associated proteins (Cas) system has opened a new window of applications in the field of gene editing. Here, we review different Cas proteins and their corresponding features including advantages and disadvantages, and we provide an overview of the different endonuclease-deficient Cas protein (dCas) derivatives. These dCas derivatives consist of an endonuclease-deficient Cas9 which can be fused to different effector domains to perform distinct in vitro applications such as tracking, transcriptional activation and repression, as well as base editing. Finally, we review the in vivo applications of these dCas derivatives and discuss their potential to perform gene activation and repression in vivo, as well as their potential future use in human therapy. Keywords: CRISPR/Cas; genome editing; transcriptional regulation; dCas9 derivatives 1. Introduction In the last ten years, major breakthroughs have been made in the field of gene editing, which is the process where DNA is modified, deleted, inserted or replaced. The most recent discovery of the clustered regularly interspaced short palindromic repeats (CRISPR)/CRISPR associated proteins (Cas) system has opened up many novel opportunities as well as applications for gene editing both in vitro and in vivo. In this review, we provide an overview of the different gene editing techniques including the CRISPR/Cas system, which was discovered in 2012. After this finding, different Cas proteins were developed with unique features that allow for distinct gene editing approaches. Here, we summarize these different Cas proteins and detail their specific features. Besides the development of different Cas proteins, endonuclease-deficient Cas proteins (dCas) allow for additional applications of the CRISPR/Cas system. These endonuclease-deficient Cas proteins (dCas) can be fused to effector domains which exert additional functions such as transcriptional activation or repression, but also tracking and base-editing. These dCas effectors as well as their functions and in vivo applications will be the main focus of this perspective. Int. J. Mol. Sci. 2020, 21, 3038; doi:10.3390/ijms21093038 www.mdpi.com/journal/ijms Int. J. Mol. Sci. 2020, 21, 3038 2 of 19 Int.2. GeneJ. Mol. Sci. Editing 2019, 20 Techniques, x FOR PEER REVIEW 2 of 19 Gene editing started with the discovery of meganucleases in yeast as well as in other small organisms.Gene editing There arestarted hundreds with ofthe meganucleases discovery of whichmeganucleases each contain in ayeast recognition as well sequence as in other (homing small organisms.site) between There 14 are and hundreds 40 base pairsof meganucleases (Figure1)[ 1 ].wh Thisich each large contain recognition a recognition site ensures sequence great (homing locus site)specificity between together 14 and with40 base low pairs off-target (Figure eff 1)ects. [1]. This At the large same recognition time, the largesite ensures recognition great sitelocus is specificity also the togethermain limitation with low of meganucleasesoff-target effects. since At their the recognition same time, site the only large occurs recognition once (or sometimessite is also not the at main all) limitationin the genome of meganucleases of a given organism. since their This recognition limitation cansite beonly circumvented occurs once by(or modifying sometimes the not recognition at all) in the genomesite of the of particulara given organism. meganuclease This limitation [2], which can is time-consumingbe circumvented and by labor-intensive.modifying the recognition site of the particular meganuclease [2], which is time-consuming and labor-intensive. FigureFigure 1. 1. GeneGene editing editing techniques. techniques. Overview Overview of the of the different different gene gene editing editing designer designer nucleases. nucleases. This Thisfigure depictsfigure depictsthe initially the initially discovered discovered meganucleases. meganucleases. In addition, In addition, the zinc thefinger zinc an fingerd transcription and transcription activator- likeactivator-like effectors (TALE) effectors are (TALE) depicted, are which depicted, are fused which to areFokl fused nuclease to Fokl monomers nuclease which monomers cleave the which DNA uponcleave dimerization. the DNA upon Each dimerization. DNA-binding Each module DNA-binding is indicated module with a is different indicated color. with The a di ffmosterent recently color. discoveredThe most recently RNA-guided discovered clustered RNA-guided regularly clustered interspaced regularly short interspacedpalindromic short repeats palindromic (CRISPR)/CRISPR repeats associated(CRISPR)/ CRISPRproteins-9 associated (Cas9) system proteins-9 is illustrated (Cas9) system as well. is illustrated Black arrows as well. represent Black the arrows cleavage represent site. the cleavage site. In 1994, the zinc finger nucleases (ZFN) were discovered as the first modulated gene editing tools [3]. ZFN consist of nucleases which are coupled to certain zinc fingers which each recognize triplets of base pairs (codons; Figure 1) [4]. To increase the binding specificity, several zinc fingers are attached together (usually three) to reach a binding site of nine nucleotides (three codons). The same holds for the complementary strand, so in total an 18-nucleotide specificity is reached. These zinc fingers can be engineered in a specific way to recognize the DNA region of interest. These connected zinc fingers are then fused to cleavage domains of endonucleases (usually to Fokl nuclease monomers) [5]. The sequence Int. J. Mol. Sci. 2019, 20, x; doi: FOR PEER REVIEW www.mdpi.com/journal/ijms Int. J. Mol. Sci. 2020, 21, 3038 3 of 19 In 1994, the zinc finger nucleases (ZFN) were discovered as the first modulated gene editing tools [3]. ZFN consist of nucleases which are coupled to certain zinc fingers which each recognize triplets of base pairs (codons; Figure1)[ 4]. To increase the binding specificity, several zinc fingers are attached together (usually three) to reach a binding site of nine nucleotides (three codons). The same holds for the complementary strand, so in total an 18-nucleotide specificity is reached. These zinc fingers can be engineered in a specific way to recognize the DNA region of interest. These connected zinc fingers are then fused to cleavage domains of endonucleases (usually to Fokl nuclease monomers) [5]. The sequence in between the two ZFN binding sites is the so-called spacer region, consisting of 5–7 nucleotides where Fokl monomers form a catalytically active dimer, which cleaves the DNA, resulting in double-strand breaks [6]. The production of an efficient ZFN might require multiple rounds of re-designing and optimizations (because each of the selected ZF might affect the binding affinity of the adjacent ZFs), which is labor-intensive, time-consuming and most importantly expensive. In 2009, the transcription activator-like effector nucleases (TALEN) were developed, which function in a similar way but have increased specificity when compared with ZFN [7,8]. Transcription activator-like effectors (TALE) were discovered in bacteria and are able to bind to single nucleotides (instead of triplets of nucleotides by zinc fingers) [9]. Similar to ZFN, several TALE are attached together (i.e., 12–31 repeats per TALEN) to target the DNA sequence of interest and are fused to the Fokl nuclease monomers (Figure1)[ 10]. Similarly, the second TALEN monomer is designed for the complementary strand. These Fokl nuclease monomers will again form a catalytically active dimer in the spacer region and cleave the DNA. The procedure of producing new ZFN and TALEN is labor-intensive since for every DNA target region of interest, novel ZFN and TALEN have to be generated. In 2012, the most recent gene editing tool was discovered: clustered regularly interspaced short palindromic repeats (CRISPR) and CRISPR associated genes (Cas) [11]. The CRISPR genomic locus consists of Cas genes (which code, e.g., for the nuclease Cas9) and a leader sequence followed by repeat sequences which are flanked by spacer regions. Originally, CRISPR was discovered in bacteria and contributes to the immune defense system where foreign
Details
-
File Typepdf
-
Upload Time-
-
Content LanguagesEnglish
-
Upload UserAnonymous/Not logged-in
-
File Pages19 Page
-
File Size-