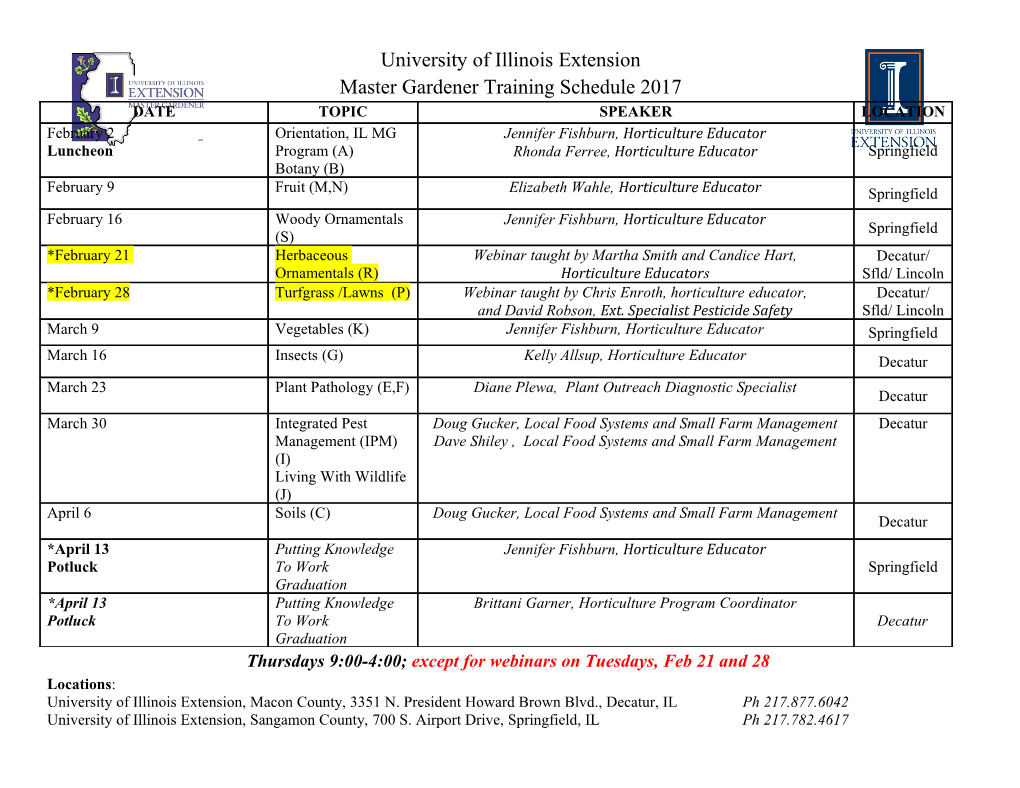
Fusion Engineering and Design 49–50 (2000) 127–134 www.elsevier.com/locate/fusengdes ALPS–advanced limiter-divertor plasma-facing systems R.F. Mattas a,*,1, J.P. Allain j, R. Bastasz b, J.N. Brooks a, T. Evans l, A. Hassanein a, S. Luckhardt c, K. McCarthy d, P. Mioduszewski e, R. Maingi e, E. Mogahed f, R. Moir g, S. Molokov h, N. Morely i, R. Nygren b, T. Rognlien g, C. Reed a, D. Ruzic j, I. Sviatoslavsky f, D. Sze a, M. Tillack c, M. Ulrickson b, P.M. Wade e, R. Wooley k, C. Wong k a Argonne National Laboratory, 9700 S. Cass A6enue, Argonne, IL 60439, USA b Sandia National Laboratory, PO Box 5800, Albuquerque, NM 87185, USA c Uni6ersity of California, San Diego, MC 0417, 9500 Gilman Dri6e, La Jolla, CA 92093, USA d Idaho National Engineering and En6ironmental Laboratory, PO Box 1625 (MS 3815), Idaho Falls, ID 83415, USA e Oak Ridge National Laboratory, PO Box 2009, Oak Ridge, TN 37831, USA f Uni6ersity of Wisconsin, 1500 Engineering Dri6e, Madison, WI 53706, USA g Lawrence Li6ermore National Laboratory, PO Box 808, Li6ermore, CA 94551, USA h Co6entry Uni6ersity, Co6entry, UK i Uni6ersity of California, Los Angeles, 44-114 Engineering IV, Box 951597, Los Angeles, CA 90095, USA j Uni6ersity of Illinois at Urbana-Champaign, 214 Nuclear Engineering Laboratory, 103 S. Goodwin A6enue, Urbana, IL 61801, USA k Princeton Plasma Physics Laboratory, PO Box 451, Princeton, NJ 08543, USA l General Atomics, PO Box 85608, San Diego, CA 92186, USA Abstract The advanced limiter-divertor plasma-facing systems (ALPS) program was initiated in order to evaluate the potential for improved performance and lifetime for plasma-facing systems. The main goal of the program is to demonstrate the advantages of advanced limiter/divertor systems over conventional systems in terms of power density capability, component lifetime, and power conversion efficiency, while providing for safe operation and minimizing impurity concerns for the plasma. Most of the work to date has been applied to free surface liquids. A multi-disciplinary team from several institutions has been organized to address the key issues associated with these systems. The main performance goals for advanced limiters and divertors are a peak heat flux of \50 MW/m2, elimination of a lifetime limit for erosion, and the ability to extract useful heat at high power conversion efficiency (40%). The evaluation of various options is being conducted through a combination of laboratory experiments, * Corresponding author. Tel.: +1-630-2528673; fax: +1-630-2525287. 1 To be presented at the Fifth International Symposium on Fusion Nuclear Technology. The submitted manuscript has been created by the University of Chicago as Operator of Argonne National Laboratory (‘Argonne’) under Contract No. W-31-109- ENG-38 with the US Department of Energy. The US Government retains for itself, and others acting on its behalf, a paid-up, nonexclusive, irrevocable world-wide license in said article to reproduce, prepare derivative works, distribute copies to the public, and perform publicly and display publicly, by or on behalf of the Government. 0920-3796/00/$ - see front matter © 2000 Elsevier Science B.V. All rights reserved. PII: S0920-3796(00)00385-9 128 R.F. Mattas et al. / Fusion Engineering and Design 49–50 (2000) 127–134 modeling of key processes, and conceptual design studies. The current emphasis for the work is on the effects of free surface liquids on plasma edge performance. © 2000 Elsevier Science B.V. All rights reserved. Keywords: Plasma; Advanced limiter-divertor plasma-facing systems; Tokamak 1. Introduction vanced limiter/divertor systems over conventional systems in terms of power density capability, com- The advanced limiter-divertor plasma-facing ponent lifetime, and power conversion efficiency, systems (ALPS) program is evaluating the poten- while providing for safe operation and minimizing tial for improved performance and lifetime for impurity concerns for the plasma. Systems being plasma-facing systems. The ALPS team has considered include both free surface liquids and worked together to address key issues through advanced solid plasma facing systems, although analysis and experimentation, to establish proce- most of the work to date has focussed on free dures for evaluation of different concepts, and to surface liquid systems. The technical information establish closer ties with the fusion plasma physics given below is limited to free surface liquid community as well as other areas of advanced systems. technology development. The main goal of the The idea of using liquids for plasma facing program is to demonstrate the advantages of ad- components goes back over twenty years [1,2] and, since then, most of the effort to examine these systems has focused on divertors in toka- maks. The liquid options can be divided into two Table 1 major classes, i.e. concepts with film flow over Possible materials, configuration, and confinement options solid surfaces and concepts with droplets or wa- terfalls. Film flow concepts are further classified Liquid species Li, Flibe, SnLi, Ga by the speed of flow and by the choice of liquid Surface Fast film, droplets, waterfall, stagnant and backing materials. Droplet concepts are fur- configuration film, pool, backside impinging jet ther classified by the droplet size, the method of Confinement Tokamak, advanced tokamak, spherical droplet formation, and the choice of liquid and options torus, field reversed configuration, backing materials. The range of options to be stellerator considered is presented in Table 1, which shows the liquids, configurations, and confinement schemes under consideration. One manifestation of the liquid surface concept is shown in Fig. 1 where liquid jets are substituted in place of solid divertor collector plates. The jet velocity is 10 m/s, and the length of the jet is 30–40 cm before entering the capture manifolds. In order to assess the potential for such liquid surface components, a number of issues must be resolved. The key issues identified are: Effect of liquid surfaces on plasma edge and core performance. Effects of transient/disruption events. Achieving high power density. DT/He trapping and release from surfaces. Fig. 1. Example of an advanced liquid surface divertor mod- The following sections summarize the progress to ule. date on addressing these issues. R.F. Mattas et al. / Fusion Engineering and Design 49–50 (2000) 127–134 129 Table 2 oration and self-sputtering needs to be further Lithium redeposition analysis with WBC code assessed. 19 The 2D UEDGE code was used to obtain Te (eV)/Ne (10 m−3) profiles of hydrogen ion density, parallel ion ve- locity, and separate ion and electron tempera- Parameter 200/1.5 tures. The base-case is an ITER-like tokamak Mean free path for sputtered atom 1.8 cm where the transport simulation sets boundary con- ionization (perpendicular to surface) Average charge state 1.8 ditions of power and density a small distance Transit time (average) 52 ms inside the magnetic separatrix and calculates the Elevation angle (average) 59° resulting scrape-off layer (SOL) profiles. Of par- Energy (average) 1 KeV ticular interest has been the effect of low-recycling Poloidal distance from launch point 7cm divertor plates such as those of liquid lithium. (standard deviation) Redeposition fraction (for 20 cm 0.99 These calculations show that the peak heat flux near-surface cut-off) actually drops in the low recycling regime, from 75 to 45 MW/m2, because electron heat transfer is in the sheath-limited regime where there is only 2. Effect on plasma edge and core performance a small drop between the midplane and the diver- tor plate. Plate electron temperature is 220 eV Interactions of the liquid surface with the for the anomalous radial transport coefficient of plasma edge will affect the operating limits of free 0.33 m**2/s for density and 0.5 m**2/s for elec- surface liquid systems. Areas being addressed in- tron and ion energy. clude: (1) surface temperature, plasma edge tem- Redeposition of sputtered particles was ana- perature, and heat flux limits on flowing liquid lyzed for the low recycling divertor using the surface divertors; (2) sputtering and evaporation WBC code [5]. For the WBC code lithium analy- effects on scrape-off layer (SOL) and edge sis, sputtered Li atoms were launched from the plasma, tritium codeposition; (3) surface transient divertor surface into a spatially-unvarying near- response to overheating; (4) helium and D–T surface plasma characterized by high electron uptake in the plasma facing surfaces, and effects temperature and low electron density. Particles on core plasma and reactor performance; (5) com- are launched with a ‘cosine’ type angular distribu- patibility between wall and divertor materials; and tion, and random-collision-cascade energy distri- (6) critical data needs (e.g. self-sputtering yields). bution, and with a preliminary binding energy estimate. The 3D particle trajectory is then com- 2.1. Modeling studies puted with a full kinetic treatment, including Lorentz force motion and charge-changing and Calculations were made to provide calculations velocity-changing collisions with the plasma. A of the 2D edge-plasma profiles in the presence of particle history terminates upon redeposition to liquid divertors and walls using the UEDGE the surface or leaving the 20 cm near-surface transport code [3,4] for the purpose of assessing region. Table 2 shows selected key redeposition impurity influx into the core plasma from evapo- parameters from this study for the case of an edge ration and sputtering from liquid surfaces. The temperature of 200 eV. The following is ob- studies to date have been in a tokamak geometry. served: (1) very high near-surface lithium redepo- The use of a hydrogen-absorbing divertor mate- sition rate (100%); (2) high redeposited average rial such as liquid lithium results in a low-recy- energy with highly oblique Li ion impingement.
Details
-
File Typepdf
-
Upload Time-
-
Content LanguagesEnglish
-
Upload UserAnonymous/Not logged-in
-
File Pages8 Page
-
File Size-