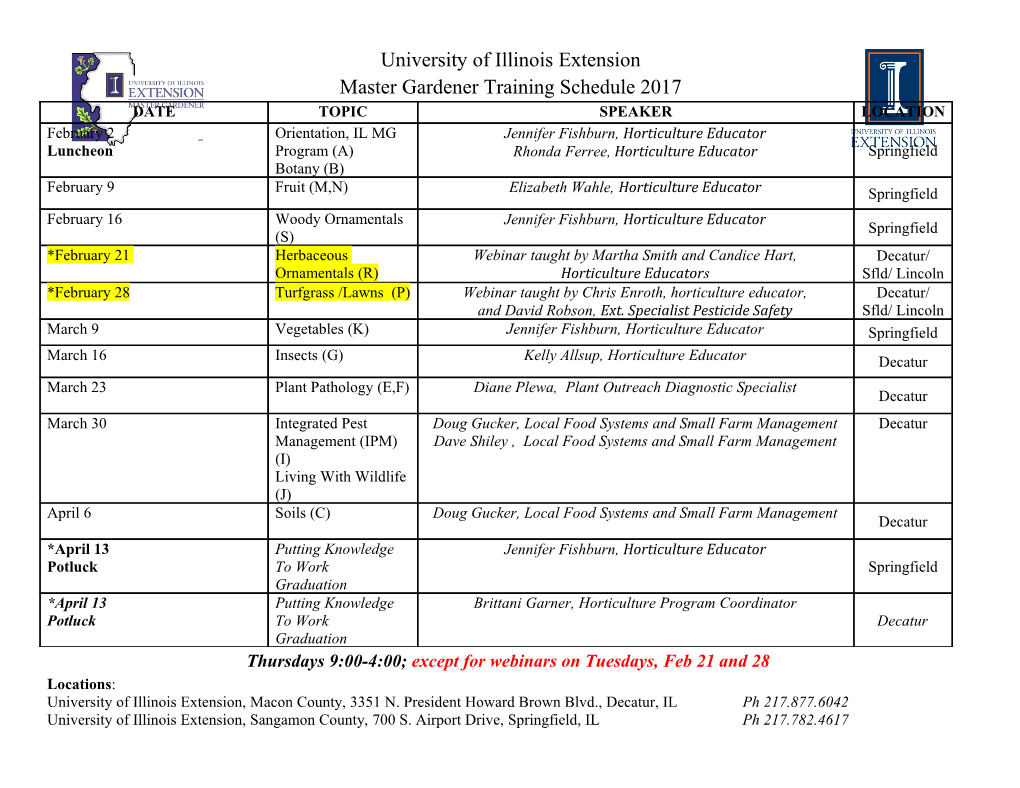
AZIDE FUNCTIONALIZATION OF CARBON MATERIALS FOR THE IMMOBILIZATION OF MOLECULAR ELECTROCATALYSTS A DISSERTATION SUBMITTED TO THE DEPARTMENT OF CHEMISTRY AND THE COMMITTEE ON GRADUATE STUDIES OF STANFORD UNIVERSITY IN PARTIAL FULFILLMENT OF THE REQUIRMENTS FOR THE DEGREE OF DOCTOR OF PHILOSOPHY Eric Dean Stenehjem May 2014 © 2014 by Eric Dean Stenehjem. All Rights Reserved. Re-distributed by Stanford University under license with the author. This work is licensed under a Creative Commons Attribution- Noncommercial 3.0 United States License. http://creativecommons.org/licenses/by-nc/3.0/us/ This dissertation is online at: http://purl.stanford.edu/kk045gz8587 ii I certify that I have read this dissertation and that, in my opinion, it is fully adequate in scope and quality as a dissertation for the degree of Doctor of Philosophy. Daniel Stack, Primary Adviser I certify that I have read this dissertation and that, in my opinion, it is fully adequate in scope and quality as a dissertation for the degree of Doctor of Philosophy. Christopher Chidsey I certify that I have read this dissertation and that, in my opinion, it is fully adequate in scope and quality as a dissertation for the degree of Doctor of Philosophy. Edward Solomon Approved for the Stanford University Committee on Graduate Studies. Patricia J. Gumport, Vice Provost for Graduate Education This signature page was generated electronically upon submission of this dissertation in electronic format. An original signed hard copy of the signature page is on file in University Archives. iii Abstract Development of molecular electrocatalysts for the efficient interconversion between stored chemical energy and electrical energy would provide a pathway towards a sustainable energy future. For use of a molecular electrocatalyst, covalent immobilization at an electrode surface is highly advantageous to provide fast electron transport and prevent catalyst loss. The copper-catalyzed azide-alkyne cycloaddition (CuAAC) reaction is desirable for such immobilization as it is selective, modular, and high yielding. Use of carbon materials for immobilization leverages their prevalent use as electrodes for energy applications. Chapter 2 develops a novel preparation of gaseous iodine azide and subsequent use for the azide modification of glassy carbon surfaces. Generating dilute gaseous iodine azide in a nitrogen carrier gas stream from the flow of iodine monochloride vapor over a column of sodium azide provides a safe and convenient method to prepare, handle, and use this potentially explosive reagent. Its immediate use to treat hydrogen terminated glassy carbon was highly reproducible and chemically specific for azide functionalization to produce surfaces containing azides as the sole nitrogen species with coverage of 3.4 × 1014 molecules cm-2, ca. a quarter of a densely packed azide monolayer. Coupling ethynylferrocene to azide-modified glassy carbon via a CuAAC reaction formed a 1,2,3-triazole linker with a coverage of 8 × 1013 molecules cm-2, a third of a densely packed ferrocene monolayer. Using X-ray photoelectron spectroscopy, the 1,2,3-triazole linker was observed to be hydrolytically stable in aqueous 1 M HClO4 or 1 M NaOH for at least 12 h at 100 °C. iv Chapter 3 expands the gas-phase azide functionalization methodology towards high surface area mesoporous Vulcan XC-72R carbon powder. The increased surface area necessitated improving generation of gaseous iodine azide to maximize yields reaching the carbon surface. A 6-fold improvement in iodine azide yield was achieved by reducing decomposition, likely due to trace water, by thoroughly drying and maintaining anhydrous conditions. Treatment of XC-72R with gaseous iodine azide results in highly reproducible and chemically specific azide functionalization to produce surfaces containing azides as the sole nitrogen species with coverage of 1.4 × 1014 molecules cm-2. Coupling ethynylferrocene achieved coverage of 1.6 × 1013 molecules cm-2. Quantitative X-ray photoelectron spectroscopy indicates that all ferrocene molecules are bonded through a 1,2,3-triazole linker with no detectable physisorbed species. Chapter 4 applies azide-modified surfaces in the development of an immobilized ruthenium electrocatalyst for the oxidation of benzyl alcohol and methanol with a 550 mV vs. NHE catalytic onset potential, a significant attenuation in potential (>300 mV) from reported immobilized ruthenium electrocatalysts. This electrocatalyst exhibits fast reaction kinetics with a TOF greater than 10 s-1 and is robust with 700 2-electron turnovers. The active catalytic species is postulated to be an IV immobilized [Ru (ethynyl-TPA)(=O)Cl](PF6), formed electrochemically in two II successive proton-coupled electron transfer steps from a Ru (OH2) species. The II surface Ru (OH2) species was generated by photoinduced ligand exchange of DMSO II on an immobilized [Ru (ethynyl-TPA)(DMSO)Cl](PF6) complex with H2O. v Acknowledgments I would like to express my thanks and gratitude to my advisor Professor Dan Stack for the opportunity to pursue my doctoral studies. The freedom and independence Dan afforded me along with his advice and guidance has provided me the opportunity to grow as a researcher. Professor Chris Chidsey has also been a valued mentor and collaborator who’s analytical and thoughtful approach to scientific problems has greatly contributed to this work. I’d also like to thank Professor Edward Solomon for acting as a reader on my thesis committee along with Professor Jennifer Wilcox for acting as the chair of my thesis defense and Professor Matt Kanan as a non-reader member. Over the course of my graduate studies I have had the privilege of working with many different colleagues, both at Stanford and other institutions. Current and former members of the Stack and Chidsey group have been a pleasure to work with on a daily basis and have each contributed their individual insights and knowledge both to my research and my growth as a scientist. I’d like to express my gratitude to all of them: Cooper Citek, Thomas Cook, Sam Fretz, Dr. Brannon Gary, Olivia Hendricks, Chris Lyons, Ross Moretti, Srinivasan Ramakrishnan, Matthijs Van den Berg, Mithi Adhikari, Paul Alperin, Dr. Katharina Butsch, Dr. Ali Hosseini, Dr. Peng Kang, Dr. Bolin Lin, Dr. Randy Lowe, Dr. Charles McCrory, Dr. Soushi Miyazaki, Dr. Jun Nakazawa, Dr. Matt Pellow, Dr. Jonathan Prange, Dr. Brian Smith, Dr. Tim Storr, Dr. Andrew Thomas, Dr. Pratik Verma, and Dr. Vadim Ziatdinov. My collaborations with researchers at GE Global Research, Lawrence Berkeley National Lab, and Yale vi University has also exposed me to individuals and organizations with diverse backgrounds that have been invaluable for my growth as a scientist. In addition, the analytically resources and expertise on campus have been invaluable to the completion of my dissertation work. Specifically I’d like to thank Chuck Hitzman, Doug Turner, and Guangchao Li for their advice in developing and troubleshooting analytical techniques. I have made many great friends during my time in graduate school and have learned much from them. I thank each of them for their friendship and look forward to continuing our friendship into the future. Finally, I am deeply grateful for the love and support of my family. Their encouragement and advice, particularly throughout the last year of my graduate studies, has helped me overcome many struggles and challenges I never imagined encountering. I owe a very important debt to them and it is to them I dedicate this work. vii Table of Contents Chapter 1. Surface Modification Methodologies of Graphitic Carbon Applicable for Covalent Immobilization of Molecular Electrocatalysts ................................... 1 Abbreviations ............................................................................................................. 2 1.1 Introduction ....................................................................................................... 3 1.2 Chemical Structure of Graphitic Carbon Materials ........................................... 4 1.3 Surface Modification Methodologies ................................................................ 5 1.3.1 Oxidation ................................................................................................................ 5 1.3.2 Aryl Diazonium Reduction ..................................................................................... 7 1.3.3 Alkenes and Alkynes Addition ............................................................................... 8 1.3.4 Carboxylate Oxidation .......................................................................................... 10 1.3.5 Chloromethylation ................................................................................................ 11 1.3.6 Azide Modification for the Cycloaddition of Alkynes ......................................... 12 1.4 Conclusion ....................................................................................................... 14 1.5 References ....................................................................................................... 14 Chapter 2. Gas-Phase Azide Functionalization of Glassy Carbon ....................... 20 Abbreviations ........................................................................................................... 21 Abstract ..................................................................................................................... 22 2.1 Introduction ......................................................................................................
Details
-
File Typepdf
-
Upload Time-
-
Content LanguagesEnglish
-
Upload UserAnonymous/Not logged-in
-
File Pages117 Page
-
File Size-