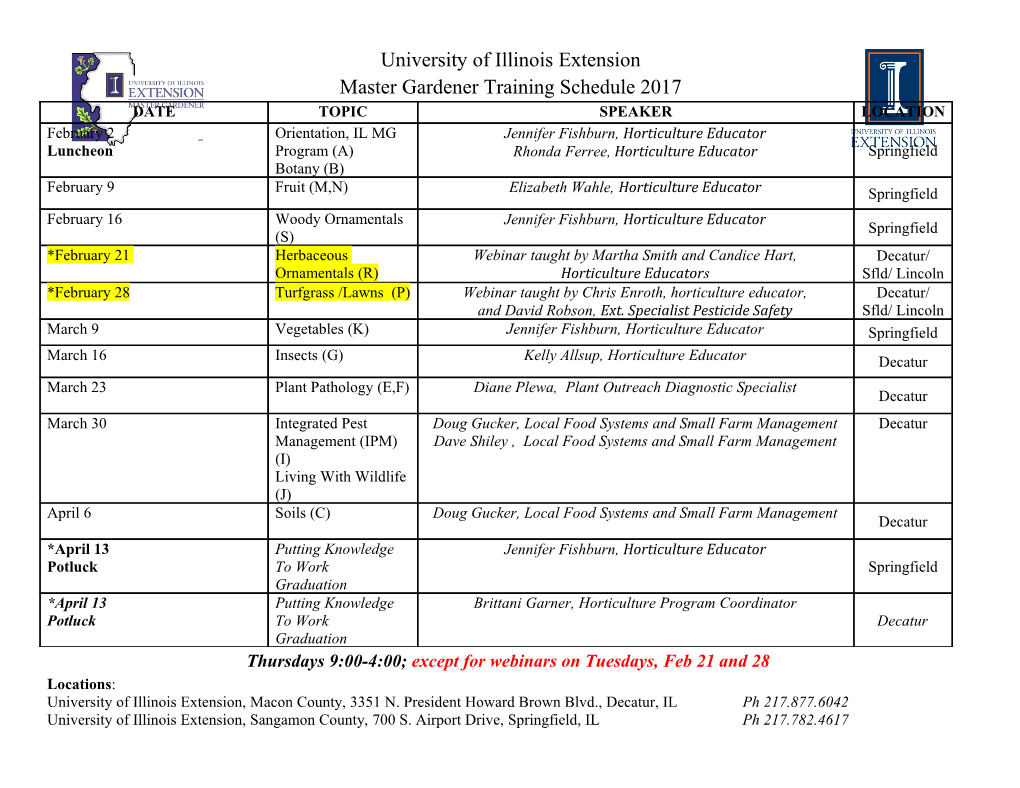
The spin Hall effect in a quantum gas M. C. Beeler*, R. A. Williams, K. Jimenez-Garcia, L. J. LeBlanc, A. R. Perry, I. B. Spielman Joint Quantum Institute, National Institute of Standards and Technology and Department of Physics, University of Maryland, Gaithersburg, MD 20899, USA Introduction to spin Hall effect Our system Spin currents Vector potential gradient • Spin Hall effect is separation of Vector potential is electron spins perpendicular to current proportional to flow coupling strength (intensity) • No external magnetic field needed – spin-orbit coupling drives effect Adjust equilibrium Current Flow Current position of BEC along • Effect is integral for spintronic devices Gaussian intensity and topological insulators gradient of Raman Color indicates predominant spin composition.1 beams 87 • Rb atoms in F = 1 ground state, confined in 90° cross-beam This is the first observation of the spin Hall effect in a optical dipole trap (ODT) Snapping off Raman cold atom system. beams gives electric • λ =790.21 nm Raman beams (Δω/2π = 15 MHz) couple mF = 0 field kick Spin-Dependent Lorentz Force and mF = -1 spin states, with large bias magnetic field along ez In intensity gradient, Lorentz Force • Adjustment of acousto-optic modulator frequency allows kick is spatially 퐹 = force dynamic control of BEC y-position by displacing one ODT beam dependent, shearing 퐹 = 푞 v × 퐵 q = charge the cloud after 2 v = velocity • No crystal structure, but system has spin-orbit coupling expansion • Cross product means that 퐵 = magnetic field particles always move • Atoms play the role of electrons, with spin coupling to linear Shear is opposite for perpendicular to velocity and 퐵 momentum along ex two pseudo-spins field. Start with equal mixture of both spins Realization of SDLF • Force proportional to charge of Measure spin current as a function of both laser coupling the particle strength and drive force Vʹ along ey (beam displacement) Abruptly change position Summary/Outlook • Particles execute curved of optical trapping beam Non-linear turn on with coupling, smoother control via trajectories gradient *Wikipedia Wait ¼ of trapping period • Spin-Dependent Lorentz Force so that atoms travel to We have realized the spin Hall effect for the first time in an center of displaced trap, Spin Transistor ultracold quantum gas 퐹 = 푠 ⋅ v × 퐵∗ 퐹 = force turn off all potentials System forms an analogue to Datta Das spin transistor3 푠 = spin • The spin Hall effect is a tool in the development of a new v = velocity Vary initial position of generation of “spintronic” devices • Elementary particles have intrinsic ∗ Drain current => spin current 퐵 = “magnetic” field atoms over small range in spin (electrons either “up” or “down”) Drain-source voltage => Vʹ (potential gradient) intensity gradient, • This system forms a prototype spintronics device – an Gate-source voltage => laser coupling strength effectively varying ey analogue to a transistor • Each spin acts as if it has opposite velocity charge response to 퐵∗ Strong similarity between black curves in top right (our • Extensions of this technique could realize the quantum spin system) and inset (characteristic transistor) Kick into and out of beams (one spin state at a time), measure Hall effect, leading to exotic topological insulators in • Lorentz-type force, but normal ∗ momentum – atoms acquire perpendicular momentum ultracold gases magnetic field does not act on spin 푩 Behind the SDLF Spin-orbit coupling Spin Current Definition Atoms with spin pointing one direction move left, atoms with In Hamiltonian mechanics, electric fields (E) and magnetic fields Define average current density: 푖 = spin ( or ) other spin move right 푛 = density 퐯= velocity (B) enter through vector (A) and scalar (φ) potentials: 퐣 = 푛 풓 퐯 풓 푑풓 /푉 풓 = position 푉 = volume 푖 푽 푖 푖 흏푨 푬 = −휵흓 − References Average spin current Average particle current 푩 = 휵 × 푨 흏풕 1Kato et. al., Science 306, 1910 (2004). 퐣 = 퐣 ′ − 퐣 ′ 퐣 = 퐣 ′ + 퐣 ′ *physicsworld.com 푠 ↑ ↓ 푝 ↑ ↓ So that the Hamiltonian (for particle of charge e) becomes: 2Lin et. al., Nature 471, 83 (2011). 3Vaishnav, J. Y., et al., PRL 101 265302 (2008). 퐣푠 = ퟎ 퐣푠 ≠ ퟎ 퐣푠 ≠ ퟎ 1 2 Ruseckas, J., et al., PRL 95 010404 (2005). This effect is usually set by a material’s properties (crystal 퐣푝 ≠ ퟎ 퐣푝 = ퟎ 퐣푝 ≠ ퟎ 퐻 = 푝 − 푒퐴 − 푒휙 Dalibard, J., et. al., arXiv:1008.5378v1 (2010). structure, doping, etc.) 2푚 Zhu et. al., PRL 97, 240401 (2006). Liu et. al., PRL 98, 026602 (2007). ∗ Our system effectively creates a vector potential 푨 acting on the Dalibard, J., et. al., arXiv:1008.5378v1 (2010). Certain materials can have the strength of SOC tuned by an spin of the particle, so that each spin acts as one type of charge *[email protected] external voltage .
Details
-
File Typepdf
-
Upload Time-
-
Content LanguagesEnglish
-
Upload UserAnonymous/Not logged-in
-
File Pages1 Page
-
File Size-